All published articles of this journal are available on ScienceDirect.
Investigation of Unsaturated Soil Hydraulic Properties for Subgrade Improvement using Marble Dust Waste
Abstract
Introduction
The distribution of suction, which has implications for seepage and shear strength, plays a crucial role in determining the stability of unsaturated soil. The Air Entry Value (AEV) and Residual Water Contents (RWC) change over time to create the Soil-Water Characteristic Curve (SWCC), which is very important for figuring out how the suction is distributed. During a flood occurrence, the subgrade becomes inundated with water, leading to adverse effects on the AEV and RWC of the subgrade. Consequently, the performance of the subgrade is diminished. This study focuses on the use of Marble Dust Waste (MDW) as an addition to the subgrade to improve the strength of the subgrade.
Aims
This study aims to investigate the variations in air AEV in relation to the optimal MDW in unsaturated soil found in Malaysia.
Methods
The study uses control samples and soil that has been mixed with different amounts of MDW: 5%, 10%, 15%, 20%, and 25%. The SWCC is generated using the pressure plate extractor device.
Results
The results indicate a significant increase in the AEV for the mixed soil sample when compared to the control sample. While the soil-MDW mixture containing 5% of this material has an AEV value of 23 kPa, the control sample's value is 10 kPa.
Conclusion
The findings of the study suggest that the utilization of MDW yields a beneficial influence on the AEV, hence potentially improving the performance of the subgrade. The researchers aim to evaluate the potentially dangerous waste and convert it into a substance that is appropriate for engineering applications. This study provides empirical evidence that aligns with the objectives outlined in Chapter 8 of the 12th Malaysia Plan for the period 2021–2025. The topic under discussion pertains to environmental sustainability, specifically focusing on enhancing the ability to withstand the adverse effects of climate change and disasters, as well as the Green Technology Master Plan Malaysia 2017–2030, with a specific emphasis on Chapter 6, which addresses the issue of waste management.
1. INTRODUCTION
Malaysia, as a tropical country, encounters recurrent instances of flooding during the monsoon season as a result of prolonged heavy precipitation and insufficient infrastructure for drainage. During and following a heavy rainfall event, water infiltrates into the slope, a part of which generates surface runoff. The change in soil structure occurrs due to a decrease in matric suction, thus affecting the cohesion between soil particles [1]. According to Hazreek et al. [2] and Ibrahim et al. [3], continuous seepage of water has the potential to undermine the stability of a slope, hence increasing the probability of slope failure. This phenomenon exhibits similarities in cases where the road infrastructure becomes submerged under flooding occurrences.
According to previous studies by Hasnayn et al. [4] and Mohd. Radzi et al. [5], the subgrade, which is the lowest stratum, experiences degradation when it is submerged in floodwater. Moisture quantity significantly influences the subgrade as well. The infiltration of precipitation into the degraded pavement and underlying subgrade has an adverse impact on the overall condition of the infrastructure [6]. In situations where the road structure becomes saturated, the presence of water has a significant impact on the performance of the subgrade. In designing pavements, determining the quality of the subgrade soil is crucial because its main function is to support the load that the layers above it transmit. According to Tey et al. [7], it is imperative for the subgrade to possess durability and stability to withstand significant traffic loads and adverse climatic conditions. Hence, it is important to implement measures to stabilize and treat the subgrade soil for road infrastructure in Malaysia, with the aim of improving their overall performance and extending their operational lifespan.
Numerous techniques are known for enhancing subgrade properties, yet there is concern regarding their cost-effectiveness and ecological sustainability [8]. Recycling solid waste has emerged as a prominent global effort to achieve the Sustainable Development Goals. An effective strategy for mitigating the buildup of waste products in the environment is the utilization of industrial wastes as alternative or supplemental materials in the replacement of natural resources [9]. Waste disposal has emerged as a significant concern in numerous countries worldwide [10]. The present study aims to investigate the potential utilization of industrial waste marble dust as an additive for enhancing the strength of the subgrade. Previous research has indicated that the optimal concentration of marble dust may vary depending on the specific characteristics of the soil. According to the findings of Jain et al. [11], there is a negative correlation between the quantity of MDW and both the liquid limit and plastic limit. The findings of Gurbuz [12] and Soosan et al. [13] provide support for the fact that the liquid limit and plasticity index of soil mixtures decline. This decline may be linked to the soil's granular properties as well as the micro-interactions between marble dust and clay.
The findings from other soil characteristic analyses indicate a positive correlation between the specific gravity of the soil and the percentage of MDW. As the proportion of marble dust in the soil increases, there is a corresponding increase in the Maximum Dry Density (MDD) and a drop in the Optimal Water Content (OWC). The MDW increases when the void ratio of the mixture decreases and the soil's gradation improves. On the contrary, a decrease in the porosity of the soil-marble dust matrix results in a drop in the OWC and a reduction in the ability to retain water. The research indicates that micro-analyses such as X-Ray Diffraction analysis (XRD) and Field Emission Scanning Electron Microscope (FESEM), as well as physiochemical assessments like pH tests, reveal significant components in soil-MDW mixes that influence their strength and swelling characteristics. The changes in the original minerals, resulting in the formation of new minerals, are accompanied by modifications in the microstructure due to improved particle gradation and enhanced interparticle bonding. Additionally, these changes also affect the original elements and shear strength characteristics.
Several countries, including India, Pakistan, Egypt, and Turkey, have undertaken research endeavours aimed at achieving subgrade stabilization through the utilization of wasted marble dust. The findings of the study indicate that the utilization of MDW, including predominantly calcium, silica, and alumina, has the potential for soil stabilization purposes. The formation of this industrial waste occurs during the marble-cutting operations conducted at quarries. The rapid growth of MDW in developing countries, accompanied by inadequate waste management practices and improper disposal methods, has led to significant environmental pollution. The present study aims to examine the potential effects of MDW as an additive in the subgrade. The use of MDW in the subgrade has the potential to address environmental concerns by reducing construction expenses and eliminating the need for waste material storage and handling [14]. According to research conducted in Pakistan, it was found that incorporating 10% MDW into the soil had several positive impacts. These include a reduction in swelling and plasticity, an increase in Unconfined Compressive Strength (UCS), and a considerable improvement in the California Bearing Ratio (CBR) [15]. According to a study conducted by Jain et al. [11] in India, it was concludedthat the incorporation of 20% MDW into black cotton soil resulted in enhanced soil strength and improved soil behaviour.
In the investigation carried out in Egypt, the researchers combined marble dust with different proportions of expansive soil samples, measured by the dry weight of the soil. Some researchers, including Abdelkader et al. [16], found that adding 15% MDW to a soil sample made the CBR value higher than in the untreated soil sample. A study conducted by researchers in Algeria employed the use of MDW to enhance the quality of soil. The findings of this study indicated that MDW exhibited potential as a stabilizing agent for soils characterized by high clay content and a well-established mineralogical composition. According to Rouaiguia and El Aal [17], the inclusion of 6% MDW in fine soil results in a significant enhancement of both soil strength and compaction parameters. The research conducted by Ural et al. [18] demonstrated enhanced soil performance, with the highest UCS value achieved by the incorporation of 5% MDW. The study conducted by Mustafa and Terzi [9] demonstrated that the inclusion of 5% MDW leads to minimal levels of swelling, shrinkage potential, and compression index. Simultaneously, this inclusion enhances the UCS and flexural strength.
Previous research has used MDW mixed with several substances, including fly ash and bentonite. In their study, Singh et al. [19] conducted a mixing process including the combination of MDW and municipal solid waste incinerator ash. In their study, Yilmaz and Yurdakul [20, 21] achieved enhanced soil performance with the incorporation of MDW in combination with bentonite. The distribution of suction during a flood event primarily affects the subgrade of unsaturated soil, which in turn affects both shear strength and seepage. The determination of the soil's shear strength characteristics is crucial in the engineering analysis and design of the subgrade. The shear strength of unsaturated soil is influenced by the matric suction. When the matric suction is below the AEV, there is an observed rise in the soil shear strength with an increase in matric suction. The negative pore water pressure induces an interparticle force that significantly enhances the shear strength of soil at a specific matric suction level. The efficacy of matric suction in reaching the point of contact between soil particles at a low water content is constrained. Consequently, the relationship between shear strength and matric suction tends to remain consistent at higher levels of matric suction.
The AEV refers to the point at which air begins to infiltrate the soil due to matric suction. The distribution of pore sizes inside the soil matrix and the maximum pore size at the soil surface are two crucial factors that have a significant impact on the determination or measurement of AEV. This is due to the fact that finer particles tend to exhibit higher AEV values. In order to gain a more comprehensive comprehension of the infiltration of water into the subgrade of unsaturated soil during a flood, it is crucial to examine the main characteristics of hydraulic properties. The AEV and RWC can be obtained by constructing a graph of the SWCC. The SWCC plays a crucial role in the analysis of the hydraulic-mechanical properties of unsaturated soil. It serves as a valuable tool for estimating many functions, including hydraulic conductivity, water storage capacity, and shear strength. SWCC plays a crucial role in the distribution of suction throughout the soil. The water retention curve depicts the correlation between the water content, denoted as θ, and the soil water potential, represented as ψ.
Previous studies have examined the impact of incorporating waste marble dust on the performance of subgrades. Nevertheless, the optimal quantity of MDW that can be obtained differs across countries, depending upon the typical soil composition in each respective research zone. Furthermore, not any investigations have been conducted to analyze the impact of the transformed subgrade's hydraulic properties, particularly the relationship between the soil's AEV and residual water content (RWC), as a result of flooding events. Hence, it is beneficial to examine and investigate the viability of utilizing waste marble dust as an addition to the subgrade for the purpose of enhancing the soil's resilience, especially in the case of flooding caused by heavy rainfall. The significance of this research consists of its relevance to the climate change phenomenon, aligning with Chapter 8 of the 12th Malaysia Plan 2021-2025, which focuses on environmental sustainability and enhancing resilience to climate change and disasters. Additionally, it supports the objectives outlined in Chapter 6 of the Green Technology Master Plan Malaysia 2017-2030, which pertains to waste management.
2. MATERIALS AND METHODS
2.1. Soil Sampling and Preparation
Soil samples were collected from several locations within a radius of the Universiti Pertahanan Nasional Malaysia (UPNM) campus situated in Bukit Gemilang, as depictedin Figs. (1 and 2). Both undisturbed and disturbed samples were collected for laboratory testing. The specimens werestored in sealed plastic bags to maintain their original state. The soil samples gathered weretransported to the laboratory and subjected to oven drying at a temperature of 105°C for 24 hours. Next, the soil sample underwentthe process of crushing and sieving to obtain a finer grain size that is suitable for future testing purposes. The basic characteristics of soil were assessed using the Unified Soil Classification System (USCS) to classify the soil. The marble waste rock comes from the GCCP Resource Limited marble quarry, which is close to Simpang Pulai, Ipoh, Perak. The waste material was subjected to crushing, resulting in its transformation into a powdered form, as demonstrated in Fig. (3). The resulting dried waste material was used as a soil sample, which was then passed through a No. 10 sieve for testing.
2.2. Soil-MDW Mixture
The soil specimens were subjected to treatment with MDW at various proportions, specifically 5%, 10%, 15%, 20%, and 25% by dry weight of soil. These treated soil samples were subsequently analyzed to ascertain the optimal MDW content. The percentages werederived from prior research conducted abroad. Table 1 presents the soil-MDW mixture design that was employed for conducting the tests.
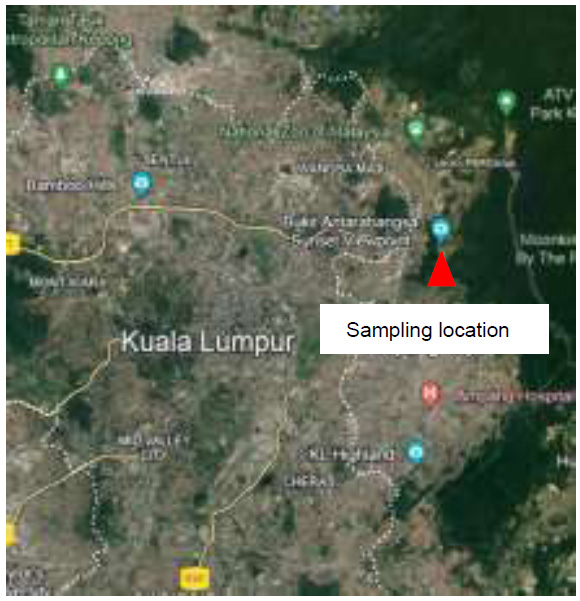
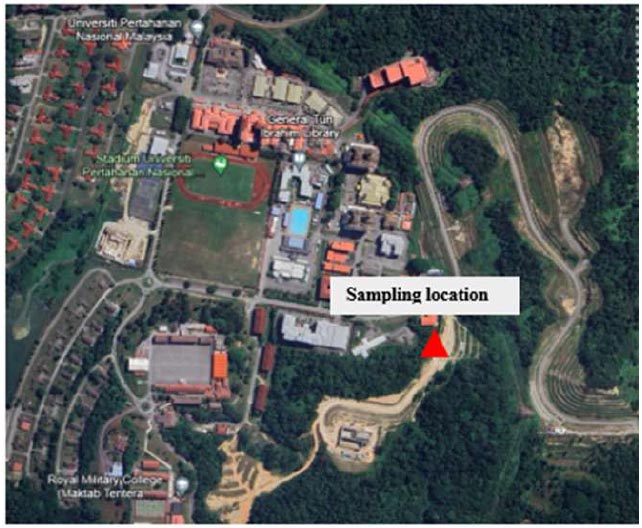
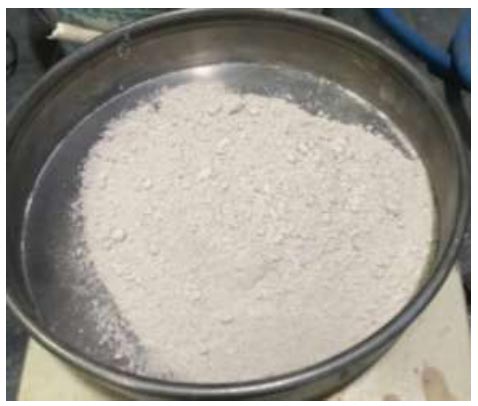
Sample Name | Soil Percentage (%) | MDW Percentage (%) |
---|---|---|
C0 | 100 | 0 |
MDW05 | 95 | 5 |
MDW10 | 90 | 10 |
MDW15 | 85 | 15 |
MDW20 | 80 | 20 |
MDW25 | 75 | 25 |
2.3. Soil Water Characteristic Curve (SWCC)
The laboratory experiments for the SWCC were conducted using the Pressure Plate Extractor Test (PPE) device. The soil sample was remoulded into a core ring with dimensions of 50 mm in diameter and 50 mm in height, maintaining the specified percentage of soil-MDW mixture. The Soil Water Characteristic Curve (SWCC) is determined in accordance with the ASTM standards of D6836-02, which specifically outline the methodology for calculating the SWCC using a pressure extractor. The experiment employs two pressure chambers, specifically a 5-bar chamber and a 10-bar chamber, along with a 20-bar air compressor and saturated ceramic plates, as demonstrated in Fig. (4). The samples, including the control sample, underwent a saturation process lasting 24 hours. Subsequently, varying levels of pressure ranging from 0 to 15 bar were administered to the samples in compliance with the standards according to the American Society for Testing and Materials (ASTM). After the application of a final pressure, the samples weresubjected to a 24-hour oven drying process, followed by subsequent weighing. The SWCC graph was generated using data obtained from the plate pressure extractor. Following that, correlation curves were produced in order to depict the relationship between the moisture content of the volume and the suction matrices. In addition, a comparative analysis was performed to assess the agreement between the empirical findings and the application of Van Genuchten's [22] equation, utilizing the R2 regression technique (Eq. 1):
![]() |
(1) |
where
Se = Normalized water content
|ϕ| = Suction pressure ([L] or cm of water)
α = Related to the inverse of air entry suction
n = Functions of the pore-size distribution
Once the SWCC graphs wereacquired, the estimation of both parameters of the AEV were conducted. Subsequently, the optimal value of the soil-MDW mixture was denoted as the estimated AEV. The analysis of results encompassed the examination and interpretation of all collected measurements, which were scrutinized and defined in order to fulfill the research objective of assessing the enhancement of AEV and RWC subsequent to a flood occurrence in unsaturated subgrade soil through the utilization of MDW waste.
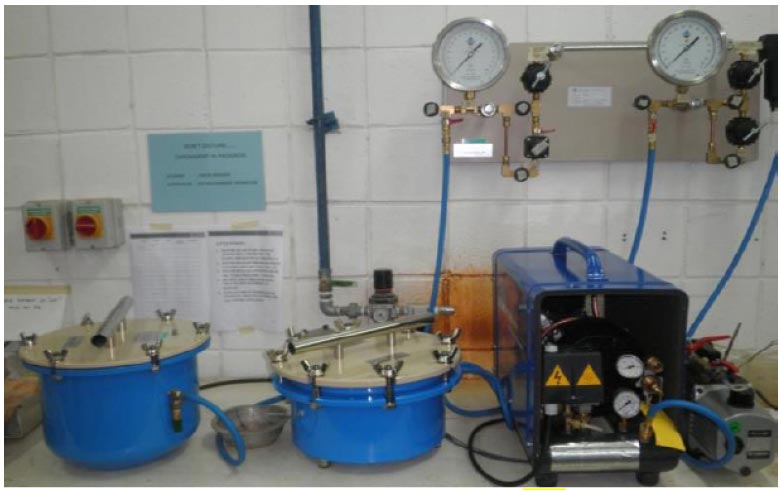
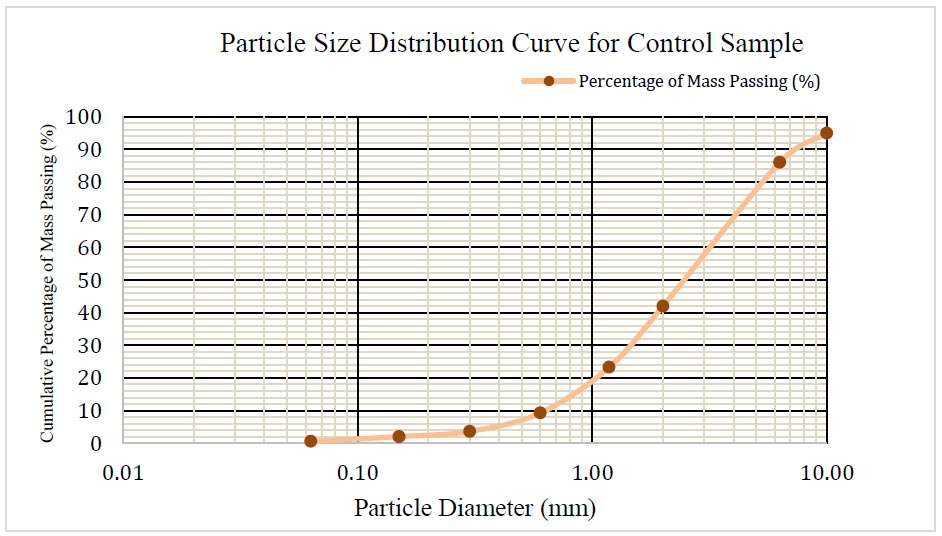
Parameter | Unit | Soil |
---|---|---|
Soil composition | - | - |
Gravel Sand Silt Clay |
% % % % |
5.1% 91.3% 3.0% 0.6% |
Soil classification | - | SP Poorly graded sand |
Hydraulic conductivity, ksat | cm/sec | 0.0124 |
Bulk density, ρb | g/cm3 | 1.64 |
2.4. Validation of SWCC using Other Equations
The Van Genuchten [22] equation, which had been frequently employed by prior scholars (Wahhab et al. [23]; Chong et al. [24]), was utilized to model the SWCC graphs. The AEV is obtained through direct crossing on the SWCC graph, and these values are correlated with the soil classification. To validate the AEV values, this study compares the AEVs calculated using the Van Genuchten [22] equation to those calculated using various equations, including the Fredlund & Xing [21] and Brooks and Corey [25] equations. Eq. (2) represents the Brooks & Corey [25] equation that was utilized to generate the fitted SWCC graph, while Eq. (3) illustrates the Fredlund & Xing [21] equation.
![]() |
(2) |
![]() |
(3) |
3. RESULTS AND DISCUSSION
3.1. Basic Soil Properties
The soil composition of gravel, sand, silt, and clay was reported as 5.1%, 91.3%, 3.0%, and 0.6%, respectively. The soil samples have been classified as poorly graded sand. The findings indicate that the bulk density of the soil is 1.64 g/cm3, as presented in Table 2. The figure demonstrated in Fig. (5) displays the particle size distribution of the control sample.
3.2. Soil-Water Characteristic Curve (SWCC)
The SWCC for the control sample is depicted in Fig. (6), whereas Fig. (7) displays the SWCC for soil-MDW mixtures. Then, the graph is fitted to conform to the Van Genuchten [22] model. Table 3 demonstratesthe result of data obtained from the fitted equation.
3.2.1. SWCC for Control Samples
The SWCC graph in Fig. (6) demonstrates that the AEV acquired for the control sample is 10 kPa. The findings of Kechik et al. [26] at the UPNM campus indicate that various sections of the Bukit Gemilang slope exhibit an AEV value of 15, which is classified as well-graded sand with gravel. In addition, the AEV value of well-graded sand with gravel and well-graded sand with silt and gravel is greater for the soil at Bukit Antarabangsa. The observed variations indicate that different types of soil correspond to distinct values of AEV. The literature has previously addressed the correlation between smaller soil particles and higher AEV values.
3.2.2. SWCC for Soil Treated with MDW
Fig. (7) illustrates the variation in AEV for the various percentages of Soil-MDW mixtures. The findings indicated an increase in AEV when varying proportions of MDW wereadded to the soil. The introduction of waste material, specifically 5% of MDW, to the control sample resulted in a substantial enhancement in the AEV for MDW05, increasing from 10 kPa to 23 kPa. The findings suggest a progressive drop in the AEV from 23 kPa to 20 kPa for MD10. The air-entry value then diminishes to 16 kPa and 15 for MD15 and MD20, respectively. However, the drop of value from 23% to 20% for MD05 to MD10 demonstrates that the real optimum value might fall within 1%-10%. Table 3 presents further information obtained from the SWCC graph for various mixtures of Soil-MDWs. The findings indicate that the volumetric saturated water content, denoted as θs, experienced a decrease across all percentages of soil-MDW. The coefficient of determination (R2) presented in Table 3 demonstrates a high level of agreement between the data derived from laboratory experiments and the equation that was fitted. This finding suggests that the incorporation of MDW into the residual soil enhances its hydraulic characteristics.
Fig. (8) presents a comparison between the control sample and the soil-MDW mixture following a 24-hour period of oven drying. The soil-MDW samples demonstrated a higher degree of brittleness when compared to the control sample, particularly for MDW15, when the AEV began to decrease, followed by MDW20 and MDW21. The modifications in the soil structure can also be observed by the change in the surface structure of the soil. Nevertheless, although the soil surface structure may be rather brittle, the higher AEV presented by the MDW suggests a significant potential for utilizing as an additive for enhancing the quality of the subgrade.
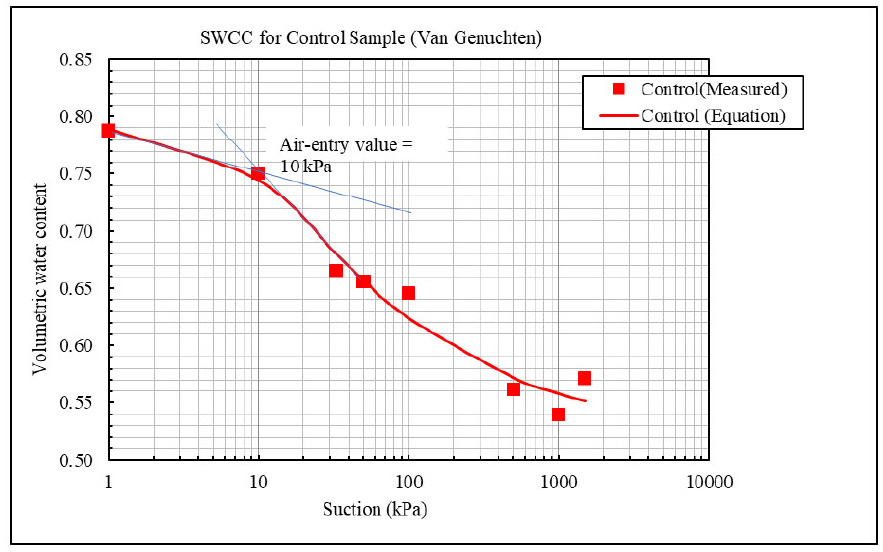
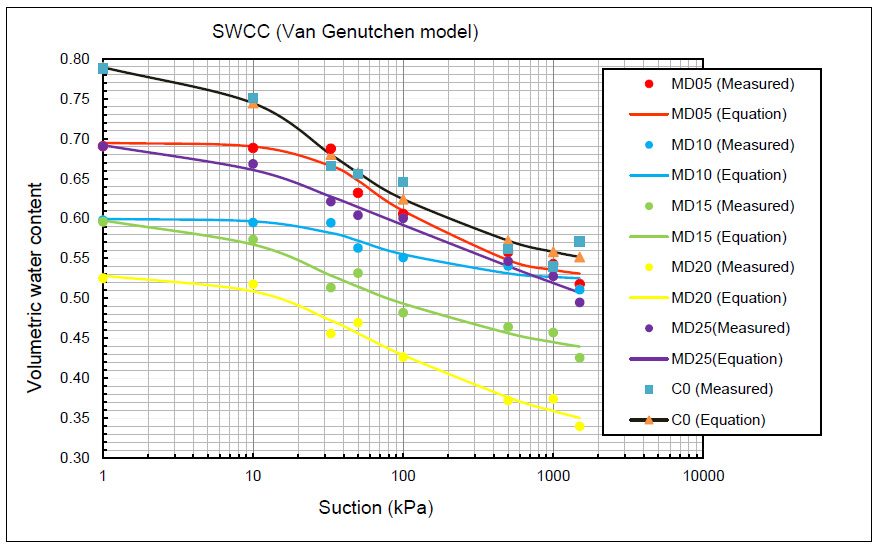
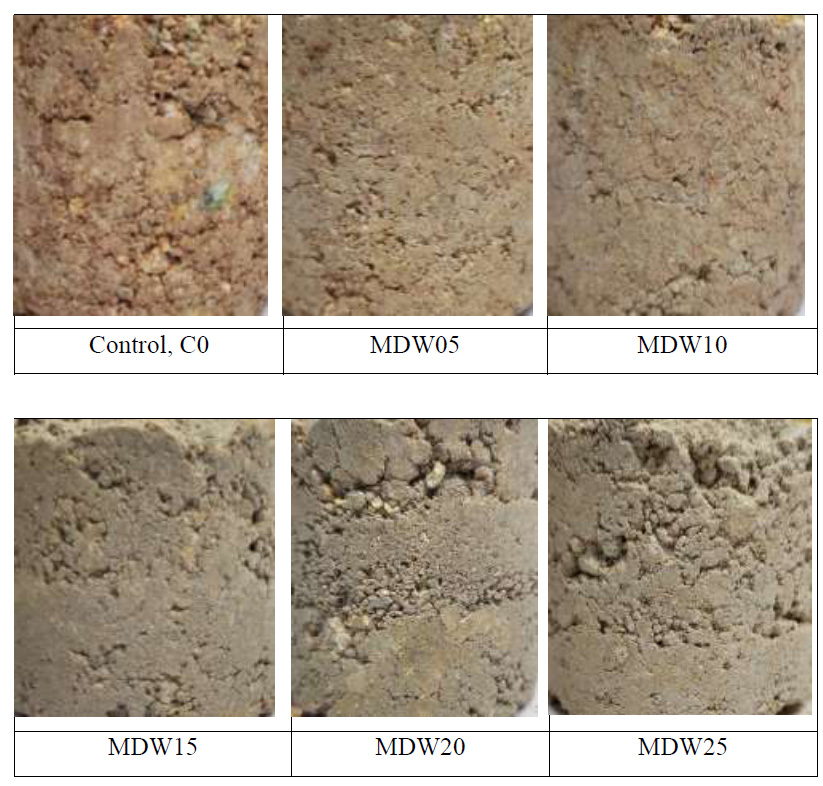
Sample Name | C0 | MD05 | MD10 | MD15 | MD20 | MD25 |
---|---|---|---|---|---|---|
Percentage of WMD | 0% | 5% | 10% | 15% | 20% | 25% |
Volumetric residual water content, θr | 0.5160 | 0.51776 | 0.52076 | 1.40E-10 | 0.27895 | 1.00E-10 |
R2 | 0.9720 | 0.9693 | 0.9111 | 0.9875 | 0.9731 | 0.9835 |
Air-entry value | 10 | 23 | 20 | 16 | 15 | 16 |
3.2.3. Validation of the AEV Values
By plotting SWCC graphs to validate the AEVs, the equations developed by Brooks and Corey [25] and Fredlund and Xing [21] were employed. The SWCC graph generated by the Brooks & Corey [25] equation is depicted in Fig. (9), whereas the SWCC graph generated by the Fredlund & Xing [21] equation is illustrated in Fig. (10). The AEVs derived from every SWCC graph are shown in Table 4. Both graphs demonstrate a good agreement for all the percentages of soil-MDW mixtures, as the R2 values are satisfactory, as stated in Table 4. In general, the analysis of the AEVs obtained from the three equations revealed that the AEVs derived from the Van Genuchten [22] equation exhibit a similarity to the AEVs mentioned in both equations.
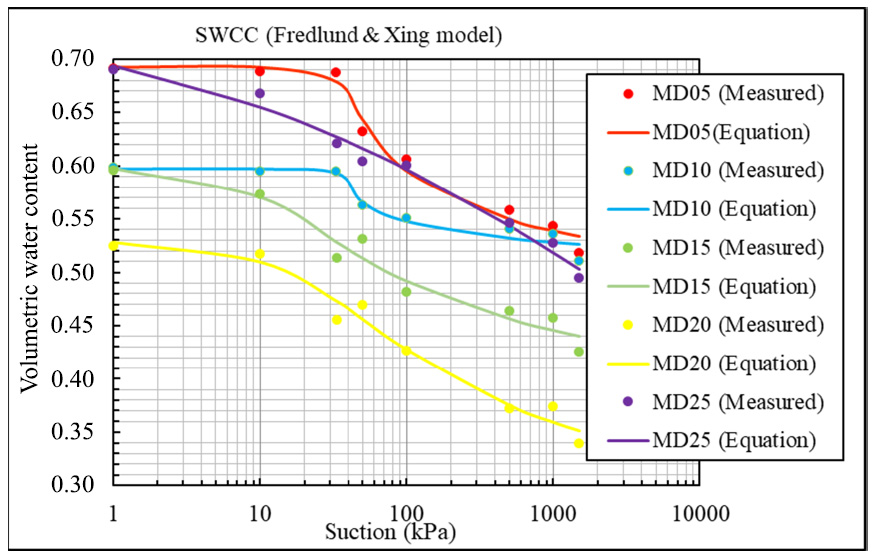
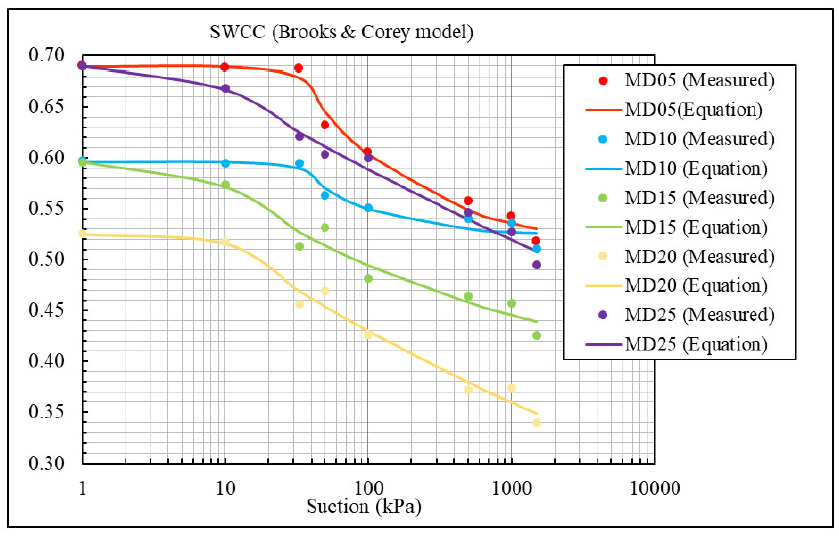
Equation | Sample Name | ||||
---|---|---|---|---|---|
- | MD05 | MD10 | MD15 | MD20 | MD25 |
AEV (Van Genuchten), kPa | 23 | 20 | 16 | 15 | 16 |
R2 | 0.9720 | 0.9693 | 0.9111 | 0.9875 | 0.9731 |
AEV (Brooks & Corey), kPa | 24 | 25 | 16 | 12 | 14 |
R2 | 0.9840 | 0.9312 | 0.9591 | 0.9770 | 0.9847 |
AEV (Fredlund & Xing), kPa | 25 | 25 | 15 | 13 | 15 |
R2 | 0.9804 | 0.9450 | 0.9569 | 0.9728 | 0.9829 |
CONCLUSION
This study demonstrated the significant enhancement of the AEV achieved by employing the SWCC for the purpose of improving the soil's condition in a submerged subgrade in the presence of rainwater. The use of MDW as an ingredient in soil mixtures leads to a significant increase in the AEV while concurrently reducing the volumetric water content. In this study, it has been demonstrated that the most beneficial soil-MDW mixture is MDW05, which consists of a 5% content of MDW in the mixture. This shows that the small percentage of MDW may increase the subgrade significantly.
However, though the AEV for MDW5 reached 23, this number decreased significantly for MDW10. Therefore, it is recommended to carry out further investigations to evaluate the soil-MDW combination within the specified range of 1% to 10% of the MDW content. In addition to this, it is necessary to perform the triaxial test and California Bearing Ratio (CBR) to assess the shear strength of the soil-MDW mixture. FESEM is recommended to be used, to examine the bonding microstructure image of soil-MDW mixes
This study provides evidence that the utilization of marble dust waste material can lead to enhancements in soil hydraulic properties, hence confirming the subsequent improvement in subgrade strength. The use of waste materials can be employed to promote sustainable development, and this purpose is intricately connected to the globally implemented Sustainable Development Goal (SDG) policies.
HIGHLIGHTS
• The utilization of marble dust waste improved the stability of the subgrade in tropical regions.
• The addition of marble dust waste enhanced the hydraulic properties of soil.
LIST OF ABBREVIATIONS
AEV | = Air-Entry Value |
RWC | = Residual Water Contents |
SWCC | = Soil-Water Characteristic Curve |
MDW | = Marble Dust Waste |
MDD | = Maximum Dry Density |
OWC | = Optimal Water Content |
UCS | = Unconfined Compressive Strength |
CBR | = California Bearing Ratio |
UPNM | = Universiti Pertahanan Nasional Malaysia |
USCS | = Unified Soil Classification System |
ASTM | = American Society for Testing and Materials |
PPE | = Pressure Plate Extractor Test |
FESEM | = Field Emission Scanning Electron Microscope |
SDG | = Sustainable Development Goal |
CONSENT FOR PUBLICATION
Not applicable.
AVAILABILITY OF DATA AND MATERIAL
Locally available materials were used in this study.
FUNDING
The research is not supported by any agency. However, the authors appreciate the support from the faculty of engineering, Universiti Pertahanan Nasional Malaysia, for the laboratory equipment usage.
CONFLICT OF INTEREST
The authors declare no conflict of interest, financial or otherwise.
ACKNOWLEDGEMENTS
The writers also gratefully acknowledge the support of Universiti Pertahanan Nasional Malaysia.