All published articles of this journal are available on ScienceDirect.
Methodology of Detailed Assessment of the Seismic Hazard of The Republic of North Ossetia-Alania
Abstract
Background:
Seismic hazard assessments produced in a probabilistic form have become the most common in the world in recent decades. Thus, specifically designed for the purpose of detailed engineering and seismic works, methodology for the probabilistic assessment of the potential seismic effects of earthquakes is proposed.
Objective:
The focus of this paper is probabilistic hazard assessments for the territory of the Republic of North Ossetia-Alania.
Methods:
The technique involves a set of models to calculate the potential seismic impacts both from certain earthquakes with different magnitude and distance and from the total number of all potentially hazardous earthquakes with different magnitude and distance, taking into account their recurrence.
Conclusion:
Specifically designed for the purpose of detailed engineering and seismic works, methodology for the probabilistic assessment of the potential seismic effects of earthquakes is proposed.
Results:
The results of calculations are presented in the form of the probability distribution functions optimally suitable for subsequent seismic risk assessments, as well as various soil reactions such as seismogenic liquefaction, seismogenic landslides, subsidence, etc.
1. INTRODUCTION
Seismic hazard assessments produced in a probabilistic form have become the most common in the world in recent decades. Such assessments allow displaying more adequately the geo-seismic reality, which is characterized by a large number of uncertainties caused by the influence of various uncontrolled natural factors on this hazard, as well as by limited modern knowledge about the processes occurring in the deepest areas of the earth's crust, i.e. where the foci of earthquakes appear and seismic waves are spreading [1-3]. In this case, forecasting of seismic hazard means estimates of the total seismic potential of the area, which can be realized (in the form of one or several earthquakes) for a sufficiently long period, without specifying the magnitude and location and time of occurrence of each certain earthquake hazardous for a given area. The main predictive element in this case is the degree of possibility (or probability) of the impacts of one or another level. Such a probabilistic approach to the seismic hazard definition is implemented in the territory of the Russian Federation at the normative level upwards 2000 [4]. Currently, the seismic hazard of the territory of Russia is regulated by the document [5], which reflects the results of general seismic zoning – the Map of GSZ-97 (General Seismic Zoning). Probabilistic hazard assessments for the territory of the Republic of North Ossetia-Alania entirely are also available only in the framework of the Map of GSZ-97 [6], i.e. in the very first approximation. As outlined in the methodological manual (teaching aid) [7], this Map primarily serves the purpose of site planning on the country, republic and large areas. Too small scale of the Map, in combination with some of the methodological provisions (regulations) laid down in it [8, 9], limits its practical use for the construction design of separate (including responsible) facilities. In this regard, it is often necessary to obtain more accurate and detailed assessments of seismic hazard when solving practical problems, including in the territory of the Republic of North Ossetia-Alania (RNO-Alania). The first step in this direction could be the creation of a probabilistic map of detailed seismic zoning of the territory of the RNO-Alania that satisfies modern requirements. In this paper the first stage of this work is described - the development of an optimal methodology detailed forecasting of probable seismic impacts for the territory of North Ossetia-Alania.
2. FORMULATION OF THE PROBLEM AND DECISION MAKING
Analysis of the most famous foreign and domestic workings (elaborations) in the field of probabilistic forecasting of seismic impacts and seismic hazard [10, 11] shows that it is reasonable to build such assessments on the basis of the total probability calculation, where the conditional probability is given by the distribution function of the considered parameter of seismic impact for a single event (earthquake) with specified characteristics (magnitude, distance, mechanism, etc.), and the unconditional probability describes the uncertainty in the occurrence of such an earthquake in a given place in a specified time interval. At the same time, different authors propose very different practical implementation of such general provision.
There are also simplified schemes, where one of the probabilities (either conditional or unconditional) is taken as a unit. Then the whole probability of impact depends either only on the uncertainty in the occurrence of a given earthquake focus (the characteristics of soil motions are assumed to be deterministic or it is believed that an earthquake will necessarily occur and the whole probability depends only on the uncertainty in predicting the characteristics of seismic motions of the ground. In the first case, there is usually an underestimation of the hazard assessment; in the second case an overestimation is observed [3].
Another common limitation is the specificity of the proposed design schemes and their lack of flexibility. So, many of them are heavily oriented towards the use of one type of information (for example, defined by a rigid scheme of parameters of the seismic setting), as well as assessment of certain parameters of seismic impacts (for example, macroseismic intensity or maximum acceleration) and to a lesser extent the use of other characteristics (for example, spectra, durations, periods, time functions, etc.).
Developments where the above issues are resolved are few and often technologically complex and difficult for implementation in a mass practical application, which seems to have affected their insufficient distribution.
Inside these problems there are still a number of more specific questions: the geometric dimensions and spatial arrangement of potentially dangerous earthquake focuses are not sufficiently taken into account in calculations, which is especially important near the seismogenic zones; the influence of local ground and geomorphological conditions is either not taken into account or taken into account in deterministic form; features of recurrence curves in zones with different maximum possible magnitudes are often ignored, etc.
In connection with the abovementioned study, the present investigation put the problem to propose a modern probabilistic methodology for assessing possible seismic impacts, which allows solving effectively all of the problems in relation to the tasks of detailed seismic zoning, taking into account the seismogeological conditions of the territory of the North Ossetia and all available scientific, methodological and available initial data.
Currently, most of the works on Probabilistic Seismic Hazard Analysis (PSHA) abroad are carried out by the S.A. Cornell method [12], which is realized in various modifications in several generations of computer programs under the general name of SEISRISK. In the Russian Federation in recent years such assessments have been carried out within the framework of the technology used for GSZ-97.
At the same time, first in the USSR and then in Russia probabilistic technology for assessing seismic impacts and seismic hazards has been developed for more than 30 years especially for engineering purposes; this technology has been successfully used in Detailed Seismic Zoning (DSZ), Seismic Microzonation (SMZ), design of responsible facilities in many seismically active areas of the world (Central Asia, the Far East, the Caucasus, Taiwan North Africa, etc.).
Examples of results obtained with the help of this technology are given in the works (Development ..., 2003, 2004), some extracts from which are given in Fig. (1).
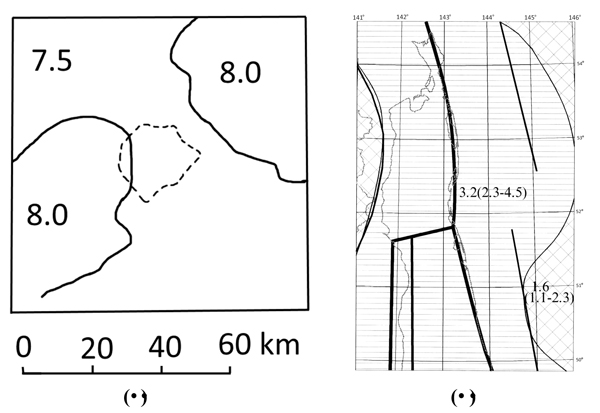
a - assessment of the probable macroseismic intensity of shakes (in MSK points) in the Pritashket district. Dotted line - borders of Tashkent city
b - assessment of probable peak accelerations (in m/s/s) in the territory of northern Sakhalin. Bold lines - the axes of the PSS zones according to V.I. Ulomov (Set of ..., 1999).
Comparative analysis of the materials described in [8-10, 13, 14] shows that the given technology has a number of advantages and is optimal for solving the tasks of DSZ of the territory of the Republic of North Ossetia-Alania taking into account the abovementioned requirements. It is also important to note that, in addition to the fact that this technology allows obtaining objectively more accurate and detailed assessments in comparison with the Map of GSZ-97, this technology at the same time is conceptually compatible with the GSZ-97 technology, which makes it possible to compare correctly the results obtained by means of this two technologies. Fig (2) shows the logic diagram of the generalized model of seismic impact, recommended in accordance with this technology for the purposes of the DSZ of the territory of RNO-Alania.
When solving the task, one should bear in mind that practical use of such complex and multiparameter models as generalized models of seismic impacts, combined with the limited initial data usually observed in practice, leads to the fact that the final results of the assessment of these impacts are subjected to fluctuations that depend on many (including subjective) factors. Such subjective factors include the choice of one or another computational scheme, selection of particular models of PSS zones, models of seismic setting, strong ground motions, selection of definite parameters of these models, etc. In fact, the integral assessment of the potential impact obtained at the output itself is only one of the possible realizations of another, more general random variable. Mechanism, of the randomness of this value is no longer formed by natural uncertainties and limited initial data (traditionally considered in the context of various probabilistic technologies (SEISRISK, GSZ-97, presented in the given paper or some other), but is formed by different concepts of certain authors about optimal methods of calculations, the choice of the design model and their characteristics, etc. And often this choice is made among alternatives almost equal in terms of their validity and reliability. In this formulation, not only the selected specific solutions, but also others “Not Selected” in each specific case alternatives actually represent the practical interest. In recent years the methodology of the “logic tree” is actively used in making the final decision for the purposes of analysis and formalized accounting of both basic and alternative options.
Therefore, it is reasonable to use the methodology of “Logical Tree” in the research on the DSZ of the territory of the RNO-Alania in seismic hazard assessment (parameters of possible seismic impacts - macroseismic intensity, peak amplitudes and spectra of soil acceleration, periods of maximum accelerations, durations of the main phase of vibrations, accelerograms etc.). This methodology makes it possible to characterize explicitly the abovementioned “subjective” uncertainties by means of direct including of alternative models and parameters into probabilistic analysis.
The final results of calculations according to the “Logical Tree” scheme are represented in the form of the most probable values of the estimated parameters of seismic impacts calculated for different levels of probability of non-exceedance. The most probable values are calculated from the total of individual alternative assessments. Certain methods of such calculations will be described in the following publications.
According to the above concept, the probabilistic analysis of seismic hazard in general consists of five main elements: construction of alternative models for the spatial location of zones of possible seismic sources (PSS zones) potentially hazardous for the investigated area; the construction of alternative models of local seismicity or the recurrence of earthquakes in time of all seismic sources that are potentially dangerous for a given section; development of alternative models of strong ground motions during potentially hazardous earthquakes, including the accounting of earthquake magnitude and distance, probabilistic analysis for each alternative model; analysis of various combinations of the results on various alternative models and their combinations.
Besides these models, the results of probabilistic and deterministic assessments of possible seismic impacts performed earlier for the investigated area (GSZ-97 Map, Building Code, etc.) are also considered as alternative assessments. A brief mathematical description of probabilistic models for calculation of possible seismic impacts and long-term seismic hazard, recommended for use for the purposes of the DSZ of the territory of RNO-Alania, is given below. More complete description can be found in [10].
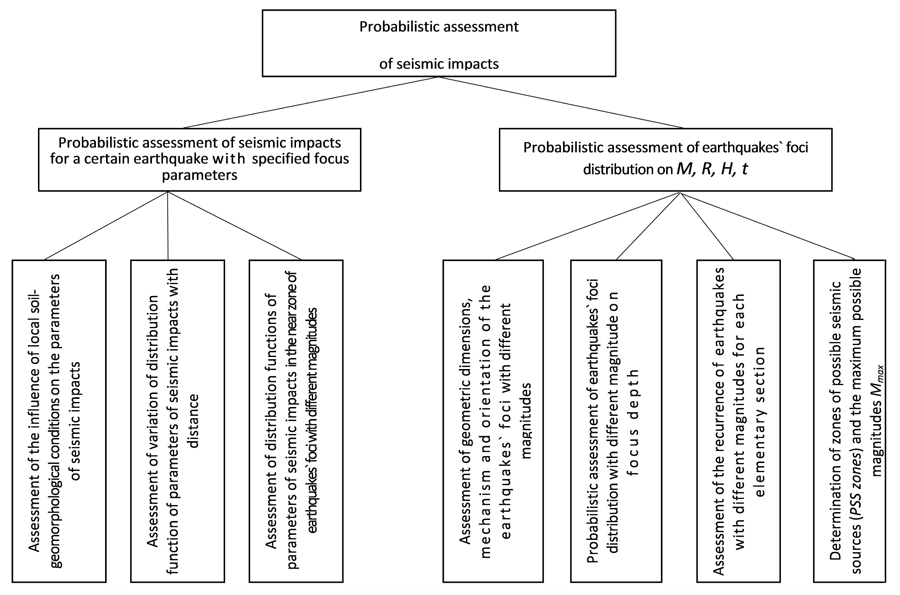
3. MODEL OF POTENTIAL SEISMIC IMPACTS FROM CERTAIN EARTHQUAKES OF DIFFERENT MAGNITUDE AND DISTANCE.
Assessments of the parameters of possible seismic impacts are given in the form of statistically assigned distribution functions of probabilities:
![]() |
(1) |
where X is a random variable (the value of the parameter of soil motions or another characteristic of the seismic impact), x is the current value of the X.
In accordance with the materials of the previous studies [8, 10] it is accepted that for the single earthquake with the magnitude M = m, the depth of the focus H = h, at a distance R = r:
![]() |
(2) |
where xi is the current value of the parameter Х; a is a mathematical expectation of the logarithm of vibration parameters:
![]() |
(3) |
where a 0 is the mathematical expectation of the logarithms of seismic impact parameters (peak acceleration of the soil – PGA, Fourier spectral acceleration – |S|, periods of peak acceleration-Т, durations of the main phase of vibration – τ) in the near zone of the earthquake; D is a distance to the source; n is the rate of change of the seismic impact parameters with distance in the far zone of the earthquake; r 0 and r' 0 are the boundaries (dimensions) of the near and intermediate earthquake zones; с1 and с 0 are coefficients of linear approximation.
The parameters а0,n, r'0, r0,с1 and с0 from the expression (3) are determined during the development of models of strong motions (attenuation functions) for the investigated area.
Expressions (1)-(3) are used for calculation of probable values lg PGA in the range of distances D = 0 – 150 km and for calculation of probable values lg |S| in the range from D = 0 to D = Dinf, where Dinf (the inflection point in attenuation curves lg |S|(D)) is assumed to be 50 km. For distances D > Dinf, the probable values lg |S| are calculated in two stages. First, according to the formula (3), the average value of lg |S| at a distance D = Dinf is assessed, then the calculation is repeated according to a formula similar to (3), with the difference that the average value lg |S| is used instead of х0, calculated for D = Dinf, - the value D = Dinf instead of the value r'0 and the rate of change of lg |S| with a distance in the interval D = Dinf – 150 km instead of the value а1.
Macroseismic intensity (I) of soil motions during an earthquake (on the 12-point scale MSK) is estimated in two ways.
The first is based on the spectral model of intensity, when the distribution function of probabilities I is calculated through the Fourier spectrum of accelerations according to the method described in the works [8-10]. There isused the variant of the technique which assumed that the I value is formed not only by vibrations at the “responsible” frequencies, but also other frequencies of the spectrum contribute in the considered frequency range. For the spectrum specified in the deterministic form, the calculation is carried out according to the formula:
![]() |
(4) |
In the expression (4), aj,f and σj,f denote, correspondingly, the values and standards of x = lg |S| assigned I = j at frequencies “responsible” for the intensity of shocks ƒj, as well as the mean values and standards for x = lg |S|, assigned to the intensity I = i,f at frequencies ƒ greater than the frequency “responsible” for seismic effect of a given force I = j; xj,f is the value of the logarithm of the observed spectrum at frequencies fj, and also at frequencies higher than the frequency “responsible” for the intensity I = j; xmin is equal to aj,f – 5σj,f as well as in the expression (4), kf is the exponent equal to the unit for the frequencies “responsible” for the macroseismic effect Ij and less than unity for frequencies greater than the frequency fj for which kf kg/ki, when kg is the empirically determined coefficient of linear regression lg |S| on I for the frequency fj.
In accordance with the recommendation [8], a simplified version of accounting for “non-responsible” frequencies is adopted in realization of calculations. For the assessment of macroseismic intensities in the range I ≤ 7 MSK points, the calculation is performed not for all the “non-responsible” frequencies fj but only for one “representative” frequency (which has the smallest total dispersion of the value lg |S|), f = 3.6 Hz, for which kf ≈0.50 is assumed.
When the vibration spectrum is given not in a deterministic form, but in the form of a set of some distribution functions of the spectral densities at the considered frequencies, the distribution functions of the shocks` intensity are given by the expression:
![]() |
(5) |
where Р[x = xj,f] is the probability that the level of soil motions spectrum at frequency fj and at frequencies “on the right” from it falls within the range of values with the center lg|S|.
This probability under the assumption that lg |S| are distributed according to the normal law, is estimated as:
![]() |
(6) |
In expressions (5)-(6), aj,f’, σj,f’are the mean values and standards for x=lg |S| preset spectral distributions at frequencies “responsible” for the effect with the load I = j and at frequencies “on the right” or greater than the frequency fj (see the expression (4). δхj,f value is equal to the half of an interval of the considered range of the values lg |S|. Usually, this value was taken equal to ≈ 0.3σj,f.
The other nomenclature in the equations (5)-(6) have the same meaning as in the equation (6). Accounting of “non-responsible” or more precisely, “less important” frequencies of the spectrum are not realized for all frequencies “on the right”, but only for one “representative” frequency f = 3.6 Hz just as in the case of deterministic task of the spectrum.
The second method is to estimate the traditional damping functions of probable values I (M, D), developed with taking into account the regional conditions of the Central and Northern Caucasus [13, 15, 16].
4. THE MODEL OF PROBABLE SEISMIC IMPACTS FROM THE TOTALITY OF ALL POTENTIALLY HAZARDOUS EARTHQUAKES WITH DIFFERENT MAGNITUDE AND DISTANCE WITH TAKING THEIR RECURRENCE INTO ACCOUNT.
The task of forecasting of possible seismic impacts is not exhausted by the estimates of the characteristics of soil vibrations from a single, particular, potentially hazardous earthquake. It is necessary to take the probability of such an earthquake occurrence into account quantitatively, and in general - the probability of the occurrence of all potentially hazardousearthquakes for a given area and for sufficiently long period of time.
Distribution functions of the parameters of seismic impacts for a single earthquake with preset values of M, H and D are given by the expressions (1-3). If the depth of the source H is precisely unknown in advance, and it can be determined only in a certain interval, then:
![]() |
(7) |
where PM=m[H = h] is the probability that the depth H of the center of gravity of the earthquake source with М= mwill be equal to h.
Earthquakes recurrence is taken into account by changing from the distribution function for one earthquake with a given M and D to the distribution function for at least one earthquake with a given M and D:
![]() |
(8) |
Here PM=m[N=n] is the probability that the number N of earthquakes with M = m in a given time interval will be equal to n. It is calculated under the assumption that N is distributed according to the Poisson's law:
![]() |
(9) |
where λm is the average number of earthquakes with M = m per unit time; t is the considered time interval, n is the expected number of earthquakes (it is chosen in the way that P[N = n] – P[N = ∞] is sufficiently small.) At a given distance for all potentially hazardous earthquakes from Mmin to Mmax:
![]() |
(10) |
And, finally, for all potentially hazardous distances from D = 0 (near zone) to D = Dmax:
![]() |
(11) |
The average period of the recurrence of impacts higher than this level can be estimated from the expression:
![]() |
(12) |
assuming that the number of such influences also follows the Poisson's law with the parameter γx. Thus parameter γx characterizes an average frequency of the excesses, and the average period of such excesses, accordingly, will be equal to the reciprocal of γx.
The computational scheme which were described above make it possible torealize calculations for point and dispersed (linear and area) objects. When assessing the seismic effect on a particular area, the investigated area is divided into quasi-homogeneous elementary regions or into elementary volumes taking into account the thickness of the seismogenic layer. Each elementary area of the territory is characterized by the following parameters taking into account the depth of the seismogenic layer:
- the values of the magnitudes of potentially hazardous earthquakes, the focus, or more precisely, the «centers of gravity» of which may arise under this area, from the minimum Mmin to the maximum Мmax;
- dimensions, mechanisms and orientation in the space of potentially hazardous earthquakes focuses with different magnitudes;
- the distribution of earthquakes with different magnitudes in the depth of the focus;
- recurrence, i.e., the average number of earthquakes with different magnitudes per unit time;
- probabilistic characteristics of soil vibrations (peak amplitudes and levels of the Fourier spectral density of accelerations at various fixed frequencies, as well as other considered dynamic and kinematic characteristics of soil vibrations) in the near zone during the earthquakes with different magnitudes from Mmin to Мmax;
- attenuation functions of peak amplitudes with a distance and Fourier spectral density, accelerations at various fixed frequencies, as well as other considered dynamic and kinematic characteristics of soil vibrations in the far zone of the potentially hazardous earthquakes with different magnitudes from Mmin to Мmax;
For the whole considered territory, the dimensions of the elementary regions on the surface of the earth are assumed to be the same and equal to 5 km×5 km, which provides sufficient detail level and accuracy of calculations for the purpose of detailed seismic zoning [9]. Assessment sare made as calculations of the cumulative impact of earthquake focus, which can occur both directly under this “Elementary” area, and under all “Elementary” areas surrounding the calculation point.
The listed characteristics are the necessary initial data for subsequent calculations. Since they are closely related to both regional and local seismicity and seismic geology of the investigated area, it is necessary to build before the calculations a number of models describing these characteristics taking into account all available seismological, geological, geophysical and engineering-geological information for the area. These models are divided into two groups: seismic activity models and models of strong ground motions. These groups of models are considered in the following publications.
The above calculation methods and their results are denoted as Variant 1 (basic computational scheme) at the next stages of the study.
5. ADDITIONAL METHODS OF SEISMIC IMPACTS` ASSESSMENT
Besides the calculation of the expected seismic impacts of Variant 1, there are provided calculations based on the recommendations of regulatory documents, as well as on the results of previously performed work, including the work on seismic hazard assessment of the considered territory.
So the intensity I of ground shocks during an earthquake is estimated according to the recommendations of the GSZ-97 Map [6] and the current Building Code [5, 17].
These (additional) assessments are also included explicitly in the formalized analysis using the “logical tree” methodology and are denoted as Variants 2, 3, etc.
In Variant 2, for example, shocks` intensitiestaken from [5, 17] can be recalculated into peak accelerations according to the ratios that generalize the recommendations of regulatory documents [6, 17]:
![]() |
(13) |
PGA (I) ratios obtained in the work [8] are also used.
![]() |
(14) |
![]() |
(15) |
recommendations from [18]:
![]() |
(16) |
![]() |
(17) |
and also the functional that we modernized from [10]:
![]() |
(18) |
In the expressions (13) - (18): I is macroseismic intensity in MSK points; PGA, amax and A is peak ground acceleration in cm/s2; Таis the period of the peak amplitudes of the acceleration of soil vibrations in c; τ0.3 and τ0.5 are duration of vibrations with amplitudes greater than 0.30 and 0.50 peak accelerations in s, correspondingly.
The ratios and recommendations from the works [19-21] can be used in the same Variant.
Variant 3 can be built, for example, on the recommendations of GSZ-97 of the territory of the Russian Federation.
CONCLUSION
Summarizing the research results, we can resume the following:
- The analysis of known methods of probabilistic assessment of seismic impacts and seismic hazard, as well as the results of detailed work performed in recent years in the Caucasus [13, 14, 22] indicates that the technology proposed by this study allows increasing accuracy and detail probabilistic models used in calculations of seismic hazard of the territory of the Republic of North Ossetia-Alania
- The second important advantage of this technique is the possibility of obtaining fairly correct estimates of the probability distribution functions of almost the entire list of parameters of seismic influences used in modern engineering seismology-macroseismic scales, peak and spectral amplitudes of accelerations, visible periods and relative durations, and accelerograms of soil vibrations.
- An additional advantage of this technique in comparison with the traditionally used methods is the use of the “logical tree” methodology, which allows reducing the uncertainties arising from the influence of the subjective factor.
- The developed methodology has enough wide possibilities for solving the tasks of the detailed seismic zoning of the territory of the Republic of North Ossetia-Alania and is optimal for today.
CONSENT FOR PUBLICATION
Not applicable.
CONFLICT OF INTEREST
The authors declare no conflict of interest, financial or otherwise.
ACKNOWLEDGEMENTS
Declared none.