All published articles of this journal are available on ScienceDirect.
Recent Advances and New Discussions on Superhydrophobic Coatings and Admixtures Applied to Cementitious Materials
Abstract
Increasing the durability of buildings is one of the biggest challenges of the construction industry of the 21st century. The problems concerning durability are usually related to the presence of humidity or to water infiltration in the porous cementitious materials used in buildings. Advances in biomimetics have allowed the development of superhydrophobic surfaces and materials, with contact angles greater than 150°, which are able to repel water and aqueous products. In this context, this work summarizes the recent advances on superhydrophobic coatings and admixtures applied to cementitious materials. Recommendations for the future improvement of such products are made. The synthesis of superhydrophobic coatings generally includes the deposition of a low surface energy material (LSEM), especially fluoroalkylsilanes, on a microroughened surface, which, in cementitious materials, is usually achieved with the help of nanoparticles or micrometric molds. In this sense, variables as the spraying time duration, and the nanoparticles concentration, surface area and average particle size were identified as directly influencing the surface superhydrophobicity. Functionalized nanoparticles can also be introduced in cement matrix during the paste mixing in order to obtain a longer lasting waterproofing effect. In this case, hybrid nanosilica may react with Ca(OH)2 through pozzolanic reaction. The C-S-H formed may incorporate the organic group of hybrid nanosilica, and might present superhydrophobicity as well, modifying the composite’s microstructure. Besides, the cost of fabricating hydrophobic materials is decisive for their market entry. Hence, the partial or total replacement of fluoroalkylsilanes with less expensive LSEMs seems promising and needs to be further explored.
1. INTRODUCTION
One of the greatest technological challenges of the 21st century construction industry concerns the improvement of durability of the buildings. Over the years, older buildings demand higher maintenance care, leading to a significant allocation of resources for conservation and recovery. Such situation can be observed in some European countries, such as Portugal and Sweden, for example [1, 2].
The durability of cement-based materials depends directly on their permeability, since the ingress of water and other harmful substances inside the concrete leads to deleterious reactions [3]. In this context, advances in biomimetics have enabled the development of superhydrophobic surfaces, whose microstructure mimics the lotus leave (Nelumbo nucifera). Such structure presents an optimized geometry and specific chemical composition, being able to repel water with contact angles greater than 150º [4]. Due to their self-cleaning potential, superhydrophobic coatings have attracted great deal of attention in both fundamental and applied research fields during recent years, including researches about cement-based materials.
Typically, the hydrophobicity of a material depends on its surface energy and microroughness [5]. A surface is characterized as hydrophobic when the water contact angle (WCA) on it exceeds 90º. Nonetheless, to reach the superhydrophobicity, the surface must present a water contact angle greater than 150º and a sliding angle (SA) lower than 10º. The sliding angle is the critical angle where a water droplet with a certain weight begins to slide down the inclined plate [6]. In smooth surfaces, low surface energy is required to reach hydrophobicity. Once null surface energy materials do not exist, microroughness is usually needed to intensify the hydrophobicity of low surface energy materials. Hence, two hydrophobic states are possible: the one following the Wenzel model and the one described by the Cassie-Baxter model adapted to surfaces with pores not filled with the deposited fluid.
In the Wenzel model, a homogeneous wettability state is reached, in which water droplets penetrate the rough grooves on the surface, intensifying its hydrophobicity (Fig. 1a) [7, 8]. In the adapted Cassie Baxter model, a heterogeneous wettability state is reached, in which air is trapped between the solid surface and the water droplet (Fig. 1b) [9-13]. Such former state usually allows reaching the highest contact angles and, thus, the superhydrophobicity.
Besides the water repellency capacity, superhydrophobic coatings on cementitious materials may also present anti-fouling [14, 15], anti-corrosion [16], anti-icing [17-20], heat-reflective [21, 22] and photoluminescence [23, 24] properties. Recently, some researchers have also explored the use of superhydrophobic admixtures in concrete, which are incorporated during the paste mixing [25-28]. This method aims to prolong the hydrophobic effect in cement-based materials, since coatings tend to lose their efficiency over time.
Although discussions around superhydrophobic surfaces applied to various materials can be found in the literature, a focused discussion on superhydrophobic cementitious materials may be missing. Hence, this paper specifically discusses the recent researches in superhydrophobic coatings and admixtures used in cementitious materials. With the aim to promote the technological improvement of such materials, research gaps are identified and recommendations for future work are made.
2. SUPERHYDROPHOBIC COATINGS APPLIED TO CEMENTITIOUS MATERIALS
Concrete is an inherently hydrophilic composite with a multi-scale roughness. Then, to reach the superhydrophobicity, its surface must undergo either a chemical and a morphological modification. These can be achieved separately or simultaneously [14].
The synthesis of superhydrophobic materials generally includes the combination of a low surface energy material (LSEM) deposition and a microroughened surface, which is usually achieved with the help of nanoparticles [14, 27, 29], micrometric molds [16, 30-32] or yet, via the nanocasting technique [33, 34]. Liu et al. [33] used the nanocasting technique, lotus leaves and polydimethylsiloxane (PDMS) to obtain a lotus-leaf-like microstructure onto the cement surface. Similarly, Horgnies and Chen [30] replicated the features of micro-pillared molds made of polydimethylsiloxane (PDMS) on Ultra High Performance Concrete (UHPC) surfaces. Arabzadeh et al. [35] used the layer-by-layer technique by applying a binding layer of epoxy followed by a layer of nanostructured superhydrophobic coating. Nonetheless, spraying or deposition of silane/siloxane-based emulsions with nanoparticles seems to be the most commonly employed method to fabricate superhydrophobic coatings on cement-based materials [21, 23, 27, 36-38].
In the above-mentioned techniques, superhydrophobicity is reached by modifying the surface chemistry and morphology separately. On the other hand, She et al. [14] proposed the simultaneous changing of these parameters. They sprayed a solution of nanosilica gel functionalized by low surface energy surfactants on concrete surfaces to achieve superhydrophobicity. Likewise, Zhao et al. [29] fabricated fluorine functionalized silica and suspended it in isopropanol. The solution was sprayed on a fluorine resin-coated concrete, creating a superhydrophobic layer.
The most commonly employed LSEMs for superhydrophobic cement-based materials are hybrid silanes (e.g. fluoroalkylsilanes) [31] and hybrid siloxanes (e.g. polydimethylsiloxane and polymethyl hydrogen siloxane) [14, 30, 39]. Among the nanoparticles, nanosilica is commonly used to create the hierarchical roughness on the surface. It can also exhibit high pozzolanic activity, reacting with calcium hydroxide and forming extra C-S-H, strengthening the concrete surface [40]. Fig. (2) illustrates the formation of a superhydrophobic coating on a cement paste surface. It can be noticed that the capillary voids on the surface are well coated, preventing water from entering them.
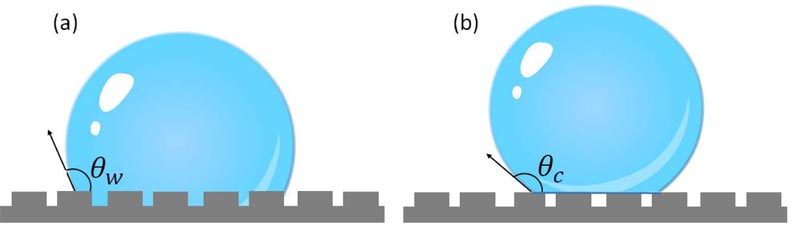
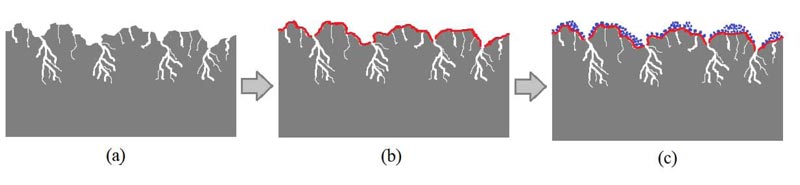
Regarding sustainability though, non-fluorinated, environment-friendly LSEMs, such as stearic acid [15, 32, 41-44], water-based stone protector (containing silane and siloxane) [16], silicone sealant [45], triethoxyoctylsilane [46] and B-doped carbon particles aggregations (BCP) [47] are very welcome on the fabrication of superhydrophobic cementitious materials. Another example of environment-friendly products is the ashes of industrial and agricultural residues. Husni et al. [37], Junaidi et al. [15], Junaidi et al. [23] used rice husk ashes, an abundantly available by-product, to create superficial microroughness to achieve superhydrophobicity. These ashes contain about 97% silica and may replace the commonly employed commercial nanoparticles in the manufacturing of hydrophobic coatings.
Some researchers proved the possibility of obtaining hydrophobic surfaces in cementitious materials only by depositing low surface energy materials on them [14, 30, 40]. However, to reach the superhydrophobicity (WCA > 150º), coatings should present a hierarchical micro-nano structure, i.e. a surface microroughness. This roughness can be achieved by incorporating nanoparticles along with the LSEMs, by incorporating functionalized nanoparticles or even by micrometric molds. Table 1 presents the results of some studies that confirm such a fact. Besides, Fig. (3) presents the wettability of modified concrete surfaces containing only nanoparticles (nanosilica), only LSEM (n-Propyltriethoxisilane – PTES) and LSEM with nanoparticles [40].
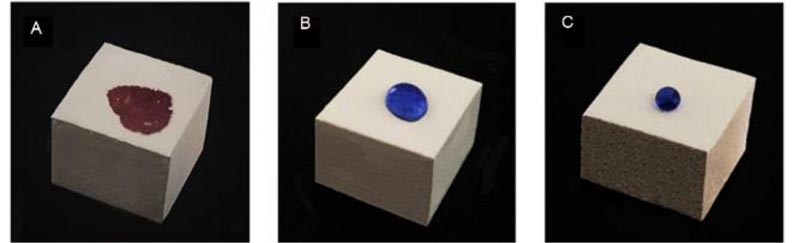
LOW SURFACE ENERGY MATERIAL | WCA (°) | SA (°) | LSEM + NANOESTRUCTURE | WCA (°) | SA (°) | REFERENCE |
---|---|---|---|---|---|---|
NP (N-propyltrimethoxysilane) | 130 | 27 | NP (N-propyltrimethoxysilane) + nanosilica in suspension | 153 | 20 | She et al., 2018 [14] |
PMHS (polymethyl-hydrogen siloxane oil) | 123 | 23 | NP (N-propyltrimethoxysilane) / PMHS (polymethyl-hydrogen siloxane oil) + nanosilica in suspension | 162 | 5 | |
PDMS(polydimethylsiloxane) | 148 | 28 | PDMS (polydimethylsiloxane) + Integrated microstructure (features of micro-pillared moulds) | 164 | 2.5 | Horgnies and Chen, 2014 [30] |
Micro-pillared PDMS (polydimethylsiloxane) | 143 | Drops stick | Micro-pillared PDMS (polydimethylsiloxane) + Integrated microstructure (features of micro-pillared moulds) | 156 | 7 |
Superhydrophobic coatings can also decrease the water absorption and permeability of cementitious materials. As stated before, these parameters are closely connected to the durability of concretes and mortars. Husni et al. [37] recorded reductions of 40.38% of the cumulative water uptake and 44.44% of water sorptivity in concrete specimens coated with a 1H,1H,2H,2H-perfluorodecyl triethoxysilane (PFDTES) and rice husk ash superhydrophobic coating. Similarly, Geng et al. [48] verified that the water sorptivity and the water uptake were reduced about 97.2% and 96.2%, respectively, in concrete surfaces coated with graphene oxide/silane superhydrophobic coatings. Superhydrophobic coatings prepared with PDMS on foundry dust and Portland cement (FD/PC) composites showed a water absorption reduction of 76% in comparison to the uncoated FD/PC composites [49].
Table 2 summarizes the development of superhydrophobic coatings on cementitious materials via different techniques.
LOW SURFACE ENERGY MATERIAL | MICRO/NANO MATERIAL | SUBSTRATE | WCA (°) | SA (°) | PROPERTIES | REFERENCE |
---|---|---|---|---|---|---|
Polymethyl-hydrogen siloxane | Metakaolin or silica fume | Concrete | 156 | - | Superhydrophobicity | Flores-Vivian et al, 2013 [36] |
PDMS (polydimethylsiloxane) | Integrated microstructure (via nanocasting) | UHPC | 164 | 2,5 | Superhydrophobicity Self-cleaning | Horgnies and Chen, 2014 [30] |
PTFE (polytetrafluoroethylene) | - | Concrete | 155 | - | Superhydrophobicity | Arabzadeh et al., 2017 [35] |
PEEK (polyether ether ketone) / PTFE (polytetrafluoroethylene) | - | Concrete | 159 | - | ||
FDTS (1H,1H,2H,2H-Perfluorodecyltrichlorosilane) | Sinalized diatomaceous earth | Concrete | 161 | - | ||
PFDTES (1H,1H,1H,2H-perfluorodecyltriethoxysilane) | Rice Husk Ash | Concrete | 152,3 | - | Superhydrophobicity | Husni et al., 2017 [37] |
PFDTES (1H,1H,1H,2H-perfluorodecyltriethoxysilane) | Rice Husk Ash | Concrete | 157,7 | - | Supehydrophobicity Photoluminescence | Junaidi et al., 2017 [23] |
PDMS (polydimethylsiloxane) | Integrated lotus leaf-like microstructure (via nanocasting) | Cement paste | 140 | - | Superhydrophobicity Self-cleaning | Liu et al., 2017 [33] |
Fluoroalkylsilane | Hydrolyzed TEOS | Luminescence Cement pavement | 152,2 | 5,4 | Superhydrophobicity Luminescence | Gao et al., 2018 [24] |
PFDTES (1H,1H,1H,2H-perfluorodecyltriethoxysilane) | Nanotitania e Nanosilica | Mortar | 162,3 | 5,7 | Superhydrophobicity | Subbiah et al., 2018 [27] |
NP (N-propyltrimethoxysilane) PMHS (polymethyl-hydrogen siloxane oil) | Nanosilica in suspension | Mortar | 162 | 5 | Superhydrophobicity Self-cleaning | She et al., 2018 [14] |
NP (N-propyltrimethoxysilane) | Nanosilica in suspension | Mortar | 153 | 20 | Superhydrophobicity Self-cleaning | She et al., 2018 [14] |
Fluorosilane | Nanosilica | Concrete | 160 | <5 | Superhydrophobicity Icephobicity | Zhao et al., 2018 [29] |
PDMS (polydimethylsiloxane) | Sandpaper (via nanocasting) | Magnesium Oxychloride Cement | 142 | <5 | Superhydrophobicity Self-cleaning | Gao et al., 2019 [34] |
B-doped carbon particle (BCP) aggregations | Natural metakaolin | Concrete | 156 | - | Superhydrophobicity Anti-corrosion | Li et al., 2019 [47] |
DMDCS (Dimethyldichlorosilane) | Fumed nanosilica particles | Concrete | 163,4 | <10 | Superhydrophobicity | Lu et al., 2019 [45] |
Commercial water-based stone protector (DC-30, containing silane and siloxane) | Nylon mesh with pore size of 300μm | Concrete | 161 | 6,5 | Superhydrophobicity Icephobicity Anti-Corrosion | Song et al., 2019 [16] |
Triethoxyoctylsilane | Microscale diatomaceous earth + sand powders | Commercial concrete testing plate | 168 | 5 | Superhydrophobicity Self-cleaning | Wang et al., 2019c [46] |
Fluoroalkylsilane | Nanotitania | Cement paste | 151,8 | - | Superhydrophobicity Heat-reflective Icephobicity Dark coloured | Zhu et al., 2019 [21] |
Silane monomer | Graphene oxide | Foamed concrete | 165,5 | - | Superhydrophobicity | Geng et al., 2020 [48] |
Stearic acid | Copper mesh with 50μm aperture, 90μm wire diameter | Concrete | 159 | 5 | Superhydrophobicity Anti-corrosion | Lei et al., 2020 [32] |
Room Temperature Vulcanized Silicone Rubber + TEOS + Dibutyltin dilaurate | - | Cement mortar or concrete materials | 151,1 | 7,8 | Superhydrophobic Self-cleaning Solar reflective | Wang et al., 2020a [22] |
10% Nanotitania | 156,5 | - | ||||
30% Nanotitania | 153,7 | - |
As stated before, the superhydrophobicity of a surface directly depends on the formation of a hierarchical topographical structure, in which water drops sit on the tip of hill-like structures leading to the presence of trapped air pockets (adapted Cassie-Baxter model) [50]. Such hierarchical structure is directly influenced by some variables that should be taken into account. One of them is the spraying time duration, which affects the coating thickness. Arabzadeh et al. [35] sprayed different coatings made of polytetrafluoroethylene (PTFE), polyether ether ketone/ polytetrafluoroethylene (PEEK/PTFE) and diatomaceous earth (DE) signalized with 1H,1H,2H,2H-Perfluorodecyltrichlorosilane (FDTS) on concrete surfaces, via the layer-by-layer method, using spray durations of 4, 6, 8 and 10 seconds. The surfaces sprayed for 10 seconds presented higher water contact angles (up to 161º) than those sprayed only for 4 seconds (up to 154º). Zhao et al. [29] verified a similar behavior when spraying a fluorosilane functionalized silica solution on concrete surfaces up to 5 times. The authors found out that, after a two-time spray, a continuous coating could be formed with a contact angle of 165.5º and a roll-off angle of 1.5º. Nonetheless, extended spraying times may dissolve the hierarchical structure, giving way to a connected solid material, which results in a decrease of the water contact angle [50].
Associated with the spraying time, variables such as the concentration, the average particle size, the surface area and even the specific gravity of the functionalized nanomaterials might affect the surface superhydrophobicity. Lu et al. [45] evaluated the influence of nanosilica (nano-SiO2) concentration on the superhydrophobicity of glasses coated with a polydimethylsiloxane (PDMS) emulsion containing nanosilica. The authors verified that, by enhancing the nano-SiO2 concentration from 0.5% to 2%, the water contact angle would increase from 155º to 169.8º. Lakshmi et al. [51] registered an increase in the WCA from 145º to 162.5º on glass surfaces with 13.71% and 16.58% of functionalized silica fume, respectively. Chen et al. [52] verified that enhancing the nanosilica concentration from 18.7% to 27.8% on a superhydrophobic solution of polystyrene (PS) and mercaptopropyltrimethoxy silane would raise the WCA from 112º to 152º. Arabzadeh et al. (2017) [35] observed that, for the same spraying time duration, silanized diatomaceous earth (DE) presented the highest superhydrophobicity when compared to PTFE and PEEK/PTFE. This could be attributed to the nanoscale porous silica-rich structure of DE, which provided a high specific surface area [53]. Additionally, the relatively bigger particle average size of silanized DE enhanced its superhydrophobicity. All these variables need to be taken into account when synthesizing superhydrophobic coatings for cement-based materials, in order to understand how mutual interactions may enable the optimization of the final coating.
The main limitation concerning the use of superhydrophobic coatings in cement-based materials lies on their durability. Factors such as coating aging and the decrease of bonding strength between the coating and the cementitious substrate, due to weathering conditions, reduce the coating effectiveness over time [54]. Besides, cracking on concrete surface may allow the entrance of water, even in coated surfaces [25]. Hence, new researches on superhydrophobic admixtures, which are introduced in the cementitious matrix during the paste mixing, have been developed in recent years. This method is called hydrophobization of cementitious material and aims to keep the waterproof property for a longer time.
3. SUPERHYDROPHOBIC ADMIXTURES INTRODUCED IN CEMENTITIOUS MATERIALS
Several authors have recently developed different methods for introducing superhydrophobic admixtures in the cement matrix in order to obtain a longer lasting waterproofing effect [25-28, 41, 55-59]. In their work, Muzenski et al. [25] created tiny air bubbles with superhydrophobic surface inside fiber reinforced concretes. The bubbles were created by mixing polymethyl hydrosiloxane (PMHS) with micro and nanoparticles of metakaolin and nanosilica, respectively. Once in the cement matrix, the PMHS molecules released small quantities of hydrogen gas, creating tiny air bubbles with controlled size. Nanosilica and metakaolin were placed on the bubbles replica surface, creating the required roughness for superhydrophobicity. Differently, Subbiah et al. [27] prepared a superhydrophobic material, comprising 1H,1H,1H,2H-perfluorodecyltriethoxisilane (PFDTES), nanosilica and nanotitania, which were introduced in the mortar matrix during the paste mixing.
Using a peculiar and less expensive approach, Wong et al. [41] partially replaced Portland cement with hydrophobic paper sludge ash (PSA) in cement pastes and concretes. The PSA is a by-product of the recycled paper industry. It was turned hydrophobic by dry ball-milling it with stearic acid, which acted as a surface functionalizing agent. Similarly, Zhu and Liao [42] fabricated a robust superhydrophobic cement block by agitating sand and cement mixture with a stearic acid-water emulsion. Besides, Dong et al. [28] synthesized a 3D porous self-cleaning superhydrophobic lightweight concrete through a combination of a liquid template (oil-in-water suspension emulsions) pore-forming method and an in situ bulk hydrophobic modification. The oil was mixed in two stages, using PDMS for modification and a volatile alkane as the pore-making agent. Microsized ceramic powders were also used to prepare a stable emulsion and to improve hydrophobic behavior. The superhydrophobic effect was confirmed by the water contact angles recorded by the authors. As examples, Zhu and Liao [42], Subbiah et al. [27] and Dong et al. [28] registered WCAs of 155.2º, 162º and 166º, respectively.
In general, the addition of superhydrophobic materials in cementitious composites also results in high water absorption and permeability reduction. Muzenski et al. [25] verified a reduction of 13.79% and 36% of these parameters, respectively. The same effect was observed by Wang et al. [56], who registered reductions of 13.5% and 10.7%, respectively. Subbiah et al. [27] registered a significant reduction of the water absorption when compared to the reference sample. As well, Wong et al. [41], verified that replacing Portland cement with 12% in mass of hydrophobic PSA reduced the water absorption, sorptivity and conductivity by 84%, 86% and 85%, respectively. It must be highlighted that the concentration of nanoparticles affects the water absorption and permeability, especially when nanosilica particles are used since the pozzolanic reaction between silica and Ca(OH)2 might take place [26, 60].
The freeze-thaw performance could also be improved with the insertion of nanoparticles. Compared to an ordinary mortar, Wang et al. [56] found out that the mass loss after 220 freeze-thaw cycles of a superhydrophobic mortar was reduced by 98.6%. Good results were also recorded by Muzenski et al. [25]. Besides, both Muzenski et al. [25] and Wong et al. [41] recorded a minimal reduction in compressive strength when comparing the superhydrophobic samples with the reference ones. However, Subbiah et al. [27] found that the compressive strength of the modified sample was 60% lower than the reference material. It is possible that the hydrophobic material could have hindered hydration reactions between cement and water [61, 62] (Fig. 4).
To avoid the loss of compressive strength, Chen et al. [26] sought to use superhydrophobic materials with pozzolanic potential. Hence, the authors prepared cement pastes with different amounts of commercial superhydrophobic nanosilica (1%, 2% and 4% per cement mass). The authors concluded that such nanomaterials react with the calcium hydroxide within the hydrated paste, forming a superhydrophobic type of C-S-H that repels water. This pioneering study turned the hydrated cement microstructure into a superhydrophobic one. Besides the water repellency capacity, the pozzolanic products increased the mechanical strength up to 71% and decreased the water sorption up to 25%.
Concerning the durability and service life, superhydrophobic admixtures seem to be more promising than coatings. The superhydrophobic lightweight concrete synthesized by Dong et al. [28] presented durable superhydrophobicity when exposed to several harsh environments, including mechanic grinding, heat treatment, and chemical erosion. Similarly, the superhydrophobic calcium aluminate cement prepared by Wang et al. [57] exhibited an extremely high mechanical stability against sandpaper abrasion under high pressure, long-time impinging with sand, repeated knife scratches, and electric-cutter cutting. According to Chen et al. [26], the addition of superhydrophobic nanosilica particles into cement pastes may increase the durability due to the pozzolanic reaction between the superhydrophobic nanosilica and Ca(OH)2 forming extra C-S-H. Moreover, Wong et al. [41] believe that the use of superhydrophobic PSA would lead to an overall improvement in concrete durability once it decreases water absorption but does not influence gaseous transport allowing the evaporation of the moisture content of concrete. This would increase the resistance to many deterioration mechanisms that require water as the reaction medium, such as carbonation or chloride ion penetration.
4. RESEARCH GAPS AND RECOMMENDATIONS FOR THE FUTURE IMPROVEMENT OF SUPERHYDROPHOBIC CEMENTITIOUS MATERIALS
Superhydrophobic cementitious materials may be a promising path to enhance the durability of buildings in the current century. In order to promote their further development, some research gaps were identified, followed by recommendations for the future improvement of the material.
As discussed in the previous sections, variables such as spraying time duration, nanoparticles concentration, average particle size, specific surface area and specific gravity might affect the superhydrophobicity of the functionalized cementitious materials, both using coating or admixture [35, 41]. Nevertheless, literature which elucidates these mutual variables’ dependencies is still lacking. Hence, for future works, we suggest studies that aim to explore how the parameters modifying the roughness of the structures, for example the nanomaterial’s specific surface area and average particle size, affect the hydrophobicity of the developed cementitious materials. In this sense, for a given nanomaterial, with a fixed specific surface area and particle size, several concentrations could be tested. Then, by varying the specific surface area and particle size values, the concentration dependency on these two variables could be plotted.
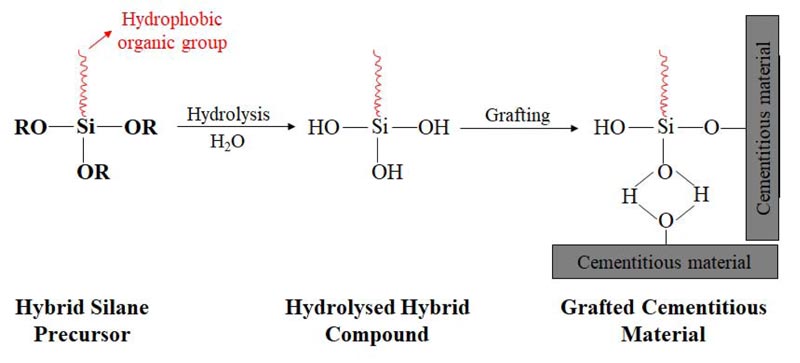
Additionally, the relationship between the hydrophobicity and the nanoparticles concentration, as well as the structural properties and the nanoparticles concentration could also be established. For this experiment, we suggest the use of sol-gel hybrid silica nanoparticles, once their specific surface area and particle size can be controlled through the sol-gel technique [63-65]. In this case, the LSEM would be used as the precursor in the sol-gel reaction. Thus, silica nanoparticles would be functionalized, presenting an inherent superhydrophobicity. These hybrid silica particles must be sprayed on the cementitious material during the sol stage, or inserted during the paste preparation, in the gel state, i. e. in the form of powder. Of course, superhydrophobic nanoparticles, preferentially dispersed in suspension to avoid the formation of agglomerates during the preparation, can be used as well. In this case, commercial nanomaterials with different specific surface area and particle size values must be acquired.
The pozzolanic reactivity of superhydrophobic nanosilica needs to be further explored since this nanomaterial can be used as a cementitious admixture. Indeed, the pozzolanic reaction of hybrid silica would simultaneously enhance both strength and hydrophobic properties of the cementitious materials, which would be an important advance for the development of structures with higher durability towards humid conditions. Based on Chen et al. [26] work, we suggest an experimental study involving superhydrophobic silica nanoparticles with different superhydrophobic functional groups, e.g. methane groups, using the Triethoxymethylsilane precursor, octane groups, using Triethoxyoctylsilane, aminopropane groups, using (3-aminopropyl)triethoxysilane, etc., introduced, using the same percentages, in cement pastes’ samples. In this case, the mechanical and structural properties of the materials with superhydrophobic extra C-S-H should be investigated. It is also expected that hybrid nanosilicas with the higher specific surface area could present faster, and probably higher, pozzolanic activity as well, so such parameter should also be considered. Moreover, as previously mentioned, the use of hybrid silica with higher specific surface area could also naturally enhance the hydrophobic property by increasing the superficial or internal roughness of the developed material.
Finally, the economic feasibility is a key factor to introduce superhydrophobic cementitious materials into the market. As –CF3 groups exhibit the lowest surface energy possible [66], fluoroalkylsilanes (FAS) are widely used in the fabrication of superhydrophobic cementitious materials. Nevertheless, such LSEMs are expensive and toxic, which might compromise the economic feasibility and large-scale production of the superhydrophobic materials as a whole. In this context, Carneiro et al. [67] prepared superhydrophobic films with 20% PFOTES (1H,1H,2H,2H-perfluorooctyltriethoxysilane) and 80% TEOS (tetraethoxyorthosilane), which is more economically feasible and less toxic than a pure PFOTES coating. They proved that such proportion could produce coatings with similar properties of those prepared with 100% PFOTES. Moreover, TEOS could be a better linker between silane/siloxane groups and the surface of the cement particles via condensation reactions [28, 56]. Fig. (3) presents the hydrolysis of a hybrid precursor, followed by its coupling with sheets of calcium silicate hydrate, resulting in the introduction of organic components into the C-S-H structure. In this sense, due to the absence of organic groups in its composition, the less expensive TEOS precursor would favor the interaction with the cement surface when used together with the hybrid one. In their work, Gao et al. [24] combined fluorine silanes and hydrolyzed TEOS to coat luminescent cement pavement materials, turning them superhydrophobic. By this method, the authors reached a WCA of 152.2º and a SA of 5.4º. Based on these works [24, 67], we suggest to further study the influence of a partial replacement of expensive and toxic precursors, e.g. FAS, by different percentages of TEOS during the sol-gel synthesis, followed by the deposition or insertion of the resulting hybrid materials in the cementitious materials, e.g. cement pastes and mortars.
CONCLUSION
A review of recent research on superhydrophobic coatings in cementitious materials indicates that it is possible to fabricate hydrophobic coatings on concrete surfaces only by using low surface energy materials (LSEMs), especially fluoroalkylsilanes (FAS). Nonetheless, to achieve superhydrophobicity, the surface must present some microroughness, which can be formed during concrete casting or via spraying or deposition of nanoparticles. The analyzed papers have prepared superhydrophobic coatings with water contact angles up to 168º and an average contact angle value of 156.8º.
To enhance the coatings durability, new researches focusing on understanding the dependency between the hydrophobic property and parameters, such as specific surface area and average particle size should be encouraged. Additionally, the relationship between the nanoparticles concentration and the hydrophobicity, as well as the nanoparticles concentration and the structural properties, should also be explored. Besides, the manufacturing cost and the toxicity degree can be key factors to the fabrication of such products on a large-scale. Hence, researches that propose a partial or total replacement of fluoroalkylsilanes by less expensive and less toxic LSEMs, without affecting the product performance, should be strongly recommended.
Finally, the pozzolanic activity of superhydrophobic nanosilica should be further investigated since it might lead to the formation of superhydrophobic extra C-S-H and the enhancement of both mechanical strength and hydrophobic properties. In such case, the hydrophobicity would be an intrinsic property of the cementitious material, and it would be expected that such material presented a longer service life.
CONSENT FOR PUBLICATION
Not applicable.
FUNDING
This work was financially supported by Centro Federal de Educação Tecnológica de Minas Gerais (CEFET-MG).
CONFLICT OF INTEREST
The authors declare no conflict of interest, financial or otherwise.
ACKNOWLEDGEMENTS
Support from CNPq, CAPES and FAPEMIG is gratefully acknowledged. This paper was designed and written by L. R. S. Carneiro and revised by M. Houmard and P. Ludvig.