All published articles of this journal are available on ScienceDirect.
Towards Zero Carbon Buildings: Overcoming the Financial Constraints to the Adoption of Biogenic Building Materials
Abstract
Introduction
The adoption of biogenic building materials is an important step towards decarbonizing the construction industry. However, a number of constraints limit their wider adoption in the industry.
Objective
To determine and analyse the financial constraints (FC) to the adoption of biogenic building materials; examine how they relate to one another; and provide strategy for overcoming the constraints to promote wider utilization of the materials in building construction.
Methods
The data for the study was obtained using methodical review of related literature and expert-based survey while the results were analysed using the decision-making trial and evaluation laboratory (DEMATEL) technique.
Results
The findings indicate that the most critical constraints with the strongest level of cause on other FC are high initial costs of materials (FC3), high design & production cost of the materials (FC4), lack of standardization & certification (FC7), difficulty in upscaling production (FC1), and limited access to capital and financing options (FC8).
Conclusion
This study contributes to advancing knowledge in sustainable construction practices by determining and understanding the specific financial constraints that impede the widespread adoption of biogenic building materials within the construction industry. By addressing these constraints, the study promotes sustainable practices within the construction sector, contributing to environmental conservation and resource efficiency.
1. INTRODUCTION
Nowadays, there is an increased interest in sustainable building solutions due to the various global environmental concerns [1]. By reducing the environmental load and using clean and renewable energy sources, it is possible to slow down the onset of environmental disaster while preserving the natural resources of the planet, without significant economic losses and changes to the existing system of relations in the field of economic activity or quality of life [2]. In order to overcome some of these environmental concerns, the construction sector adopts one of the most successful and unique approaches used in modern construction practice, the utilization of natural building materials [3]. Their reduced ecological impact, beneficial effects on human health and comfort, along with a number of further positive impacts, make their use very promising. Biogenic building materials refer to the natural building materials with low embodied energy, such as straw, reed, and wood [4]. These materials are produced from plants grown on a human timescale. One of the key differences between 'biogenic building materials' and 'renewable materials' is that renewable materials can grow back despite the fact that they have been widely used [5].
Biogenic materials, like wood, straw, and reeds, are quite common in the construction industry [6]. Along with their ecological and aesthetic benefits, biogenic and low-processing materials are a cost-effective solution for smaller buildings, such as wooden houses [7]. The wider use of biogenic materials in the construction industry involves the construction of industrial buildings or production halls from similar materials [6]. There are already a number of industrial buildings that are built from these materials, and the number of similar buildings is constantly growing [8]. However, commercial, residential, public, and other types of buildings are a rarity. This is why the use of biogenic materials in the construction of any type of building is still considered a risky or unstable novelty [2].
In recent years, biogenic materials and construction technologies have become increasingly popular, and scientific research in the field of application of bio-composites for construction is taking place in many countries [8]. However, the wide use of such materials in the construction sector is still limited for some reasons. Some of the problems are directly related to the standardization of these materials, while others are of an economic and financial nature [2]. This paper outlines the main financial barriers to the wide use of biogenic building materials together with ways to overcome these barriers. While the social, technical, regulatory, environmental, and other barriers are important considerations, the financial barriers often serve as a fundamental obstacle that affects the feasibility, scalability, and competitiveness of using biogenic materials in building construction [9]. In essence, the financial barriers are often considered more crucial than the other barriers due to their direct impact on decision-making and project feasibility. Therefore, addressing these financial challenges is crucial to accelerating the adoption of sustainable building practices and materials in the construction industry [10]. Accordingly, the research and the developed methodology can be used to stimulate the wider use of other types of innovative building materials in construction.
1.1. Novelty of the Study
The novelty of this study lies in its focused exploration of the financial constraints hindering the widespread adoption of biogenic building materials in construction. While the importance of biogenic building materials for decarbonizing the construction industry is widely acknowledged, this study stands out by focusing on the specific financial obstacles impeding their adoption. By examining the financial constraints, the study provides a deeper understanding of the challenges that are often overlooked or underestimated. The study not only identifies the financial constraints but also aims to analyse how these constraints interplay with each other. This holistic approach is crucial for devising comprehensive strategies that address the multifaceted nature of financial barriers in adopting biogenic building materials. Above all, this study has the potential to make a significant impact by facilitating the transition to zero carbon buildings through the increased adoption of biogenic materials. Its novel approach to addressing financial constraints opens up new avenues for innovation and progress in sustainable construction practices.
1.2. Overview of Biogenic Building Materials
Many modern building materials are based on natural renewable components and are created without synthetic chemical treatments [11]. Examples of biogenic building materials include straw panels, particleboard from sorghum stalks, and combined materials. Many biogenic materials are used in building, such as wood, cane strips, earth blocks, cork, expanded clay blocks, and slag blocks. Due to the increasing awareness of reducing the carbon footprint of the construction sector, biogenic materials are increasingly used [12]. The term biogenic materials refers to any type of material derived from biological sources [13-14]. More specifically, a renewable resource is a key characteristic of biogenic materials. Biogenic materials can also be smart materials that can perform multiple functions [15]. The use of biogenic building materials has developed over the past twenty years [16]. During this time, a great number of new materials based on concrete, glass, ceramics, and polymeric materials have been developed, in many cases adopting the principles of circular economy and systems thinking [17, 18].
For biogenic building materials, the raw materials utilized are based on renewable resources [19]. This applies to all the organic components and is already cost-efficient [20]. Contemporary buildings and cities as a whole can be deployed through the use of available renewable resources [2]. Analogously, appealing for future infrastructure, it can become attractive for a sustainable built environment specifically for a biorefinery [21]. Based on technological characteristics, each material can be divided into one of the following types: biomass, cellulosic substances, biopolymers, mycelium and vegetative products, food processing waste, fast-growing plants, bacteria-related products, or plants [19]. Furthermore, each biogenic building material has its own functions in the building material: structure, insulation, sealing materials, or plaster while aesthetic appearance can also be an important function and can greatly influence user acceptance [11].
2. FINANCIAL CHALLENGES IN THE USE OF BIOGENIC BUILDING MATERIALS
The increasing use of biogenic construction materials contributes to the reduction of greenhouse gas emissions, since CO2 is used in the production process from fermentation stages, and the obtained construction materials significantly reduce greenhouse gas emissions in their disposal [10]. Therefore, replacing traditional construction materials with biogenic materials is a promising environmental contribution to both current and future generations [22]. Despite making concerted efforts to introduce new types of materials for construction, the use of materials produced from biogenic materials is still relatively low [23]. This situation is associated with crucial financial challenges, primarily due to high initial cost of emerging materials, reluctance to take risks, unpredictable nature of market conditions and the lack of methodical support, along with the prevailing commercial model of use [23, 24].
The financial challenges affecting the use of biogenic building materials are directly affected by the immediate cost implications of using the materials in the construction sector [16]. The higher upfront costs tend to discourage stakeholders from selecting these materials, even if they offer long-term benefits. In addition, these financial challenges tend to further cause uncertainties around the return on investment of using the biogenic materials [1]. Thus, stakeholders may choose less expensive, conventional materials if they do not fully understand the financial advantages over a building's life cycle [11]. Often, biogenic building materials can compete on price and quality with traditional building materials, but their high production costs, lack of technologies, information about their technical and economic characteristics, and the presence of a production and sales cycle are factors that often prevent the use of biogenic materials in construction [23].
When comparing the costs of a biogenic building material with traditional building materials, not only procurement but also manufacturing and end-of-life are important. The increased effort on the input needed for the use of biogenic building materials can be related to all three [17]. The higher effort on raw materials results from the longer supply chains or the seasonal availability of renewable resources due to external influences, and the subsequently required extended handling steps for the delivery of a building material [10]. The construction of a quarterly changing, seasonal supply chain through energy crop cultivation as a raw material source for fibre production has the potential to substitute for wood-based materials in energy-absorbing products, thus expanding the scope of bio-composites as building materials [25]. Narrower supply chains, such as, the use of plant fibres, chopped straw, annual plants, and so on directly as a raw material, also, offer shortened handling paths [26]. Energy-efficient agricultural machinery mostly does the input and in order to maintain straw, rice husk, wheat hulls, etc., they also have to be further processed from time to time [27].
Not that alone, entry into the market is made more difficult by the fact that the potential adopters of such first-mover technologies have no choice but to invest the cost difference [3]. With a lack of market data and thorough knowledge of biogenic raw materials, they are likely to perceive the risk to be high, especially as it presents itself in what they know as new risk [28]. It can therefore be assumed that the demand for this type of building material will be low if the financial challenges are not overcome [9]. However, at the product level, the initial costs represent the most frequently cited financial hurdle associated with the use of building materials from renewable resources in construction activities [29]. This refers to the fact that biogenic construction materials are primarily or exclusively allocated specially and must thus be integrated at the end user level and, in the case of craftsmen, also at the intermediate user and consumer level [29]. Since biogenic building materials are usually part of only the last participant values chain, an institutional shift is envisaged [25]. The costs of re-equipping the operatives in the construction industry and training them, as well as the costs of reorientation and knowledge acquisition, are either dependently pushed back onto construction customers or serve on the side of the material user as a reason not to switch [2].
Although some of the financial constraints to the use of biogenic building materials in construction have been identified by previous studies as shown in Table 1, most of these studies did not systematically examine the interrelationships among these constraints. Overcoming the financial constraints to the wide adoption of biogenic building materials in the construction sector can be achieved by having a proper understanding of how the various financial constraints interrelate with one another. As a result, the goal of this study is to determine, analyse and prioritize these constraints and look into how they are related to one another. The study will further provide strategy for overcoming the financial constraints in order to promote the wide utilization of biogenic building materials in the construction sector. The expected findings of this article would provide significant insight into the financial constraints affecting the wide usage of biogenic building materials in construction. In essence, policymakers, decision-makers, and other important stakeholders in the construction business must have a thorough understanding of these constraints in order to develop strategies that will effectively mitigate their effects and encourage the use of biogenic building materials in the construction industry. By promoting the wider use of biogenic building materials in construction, the study generates important knowledge and evidence that might be used to promote more sustainable construction practices and contribute to the reduction of greenhouse gas emissions.
3. METHODOLOGY
A general summary of the techniques used to accomplish the study's main objectives is given in this section (Fig. 1). In order to determine the primary financial constraints to the construction industry's adoption of biogenic building materials, a thorough review of relevant literature was first carried out.
Table 1.
High initial costs of materials | [1, 9, 10, 11, 15, 16, 19, 21, 26, 28, 30] |
High design cost of materials | [6, 7, 12, 13, 15, 16, 27, 30] |
High production costs | [2, 6, 8, 9, 15, 20, 23, 27] |
Lack of technologies | [2, 7, 8, 11, 15, 17, 18, 19] |
Lack of information about the materials' economic & technical characteristics | [2, 13, 16, 20, 27, 29] |
Limited access to capital and financing options | [1, 9, 10, 23, 29] |
Market prejudice against biogenic resources | [2, 5, 14, 17, 24, 26] |
Lack of market standards | [6, 9, 15, 17, 22, 25, 26] |
High cost of certification & accreditation | [7, 8, 11, 28, 29] |
High cost of developing new standards | [6, 7, 13, 19, 25, 27] |
Reluctance to take financial risks | [2, 8, 9, 15, 23] |
Desire to import materials due to high cost locally | [7, 8, 9, 11, 26, 28, 29] |
Owners' unwillingness to use biogenic materials | [2, 6, 7, 12, 13, 19, 25, 27, 28] |
Low demand for biogenic materials | [2, 13, 16, 20, 27, 29] |
Low market awareness of the benefits of biogenic materials | [2, 6, 7, 12, 13, 19, 25, 26, 27] |
Difficulty in extending economic viability throughout the supply chain | [2, 5, 14, 17, 24, 26] |
Difficulty in upscaling production | [2, 4, 14, 17, 18, 20] |
Lack of standardization & certification | [6, 9, 15, 17, 22, 25, 26] |
Limited availability and accessibility of biogenic materials | [2, 4, 14, 17, 18, 20] |
Poor funding for research & development | [4, 16, 21, 22, 24, 29] |
Resistance of established companies to financial competition from new materials. | [2, 6, 7, 8, 13, 15, 19, 25, 27] |
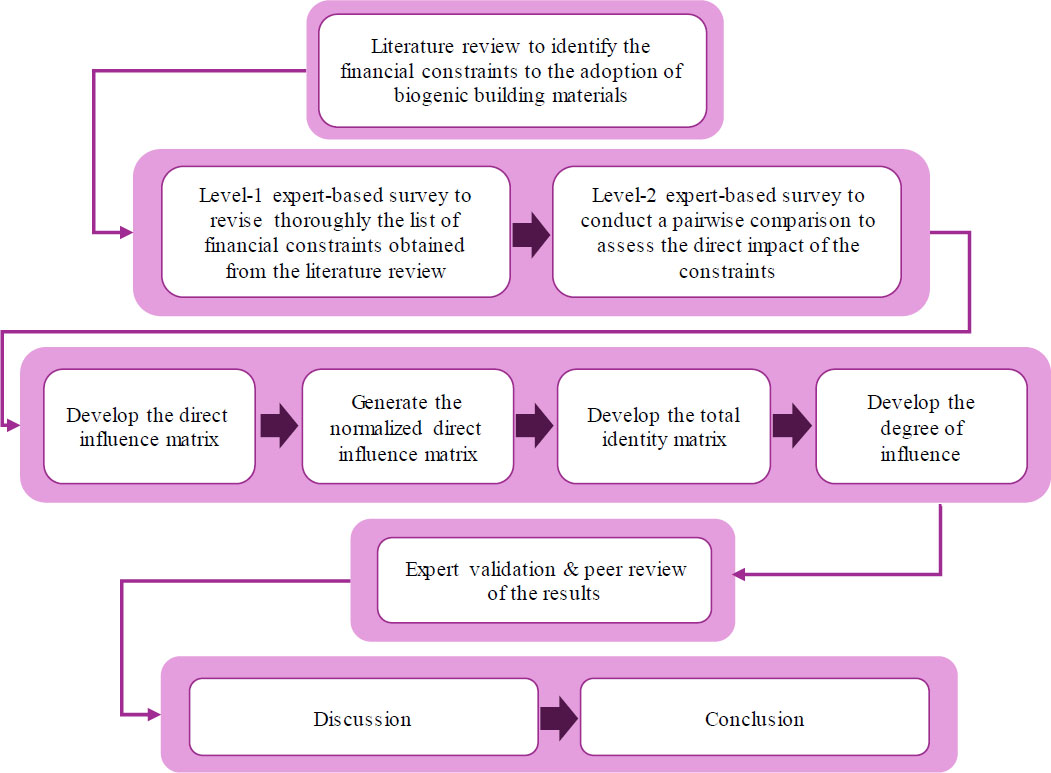
Research methodology.
3.1. The Retrieval Process for the Relevant Literature
To find peer-reviewed journal papers empirically related to this paper, a thorough desktop search was conducted using the Scopus database. Editorials, book reviews, and conference papers, for example, were excluded since these types of publications are not well examined and are not widely shared among scholars [31]. Additionally, peer-reviewed publications that are the most important, well-known, and trustworthy research findings are typically classified as “verified knowledge” [32]. Considering Scopus database’s extensive record of published journal articles and comparatively quick indexing process, which increases the likelihood of retrieving recent scientific publications related to the study, the database has been used extensively for reviewing related literature when compared to other reliable databases like Web of Science, PubMed, and Engineering Village [33].
Two sets of conditions were applied in order to identify the most appropriate articles. The first condition ensures that only published journal articles that exclusively discuss research on “sustainable building materials,” “natural building materials,” “biogenic building materials,” “biogenic construction materials,” and “bio-based building materials” were taken into account during the evaluation process. Furthermore, the second condition guarantees that the articles must primarily rely on empirical reasoning [34]. By setting these conditions, a viable list of 25 journal articles was generated, which is thought to be sufficient to identify a research gap for this type of study [35].
3.2. The Expert-based Evaluation Approach
In order to ensure the completeness, correctness, and validity of the identified financial constraints, the study opted for the effective expert-based survey evaluation approach [36]. A multi-level expert-based survey was used in this study to determine and validate the financial constraints to the use of biogenic building materials. It was determined that the best approach was to select a small number of specialists who have first-hand knowledge in implementing bio-based construction projects or developing and introducing new bio-based building materials to the market. Although there are case studies of financially and environmentally successful circular bio-based construction projects, they are not widespread or mainstream [37]. This essentially led to the selection of a smaller sample size for this study. Not that alone, it was thought that targeting specialists with comparable experience was more appropriate than expanding to a bigger sample group with less experience, authority, and knowledge [38]. Thus, in order to obtain judgments from a variety of viewpoints, it was made sure that specialists were selected to include academic researchers, architects & design engineers, construction industry professionals, financial & economic analysts, manufacturers & marketers of biogenic building materials, personnel of stand- ardization organization, and sustainability experts from both the private and public sectors.
Initially, a rigorous judgmental selection procedure was used to carefully choose 28 industry experts in a variety of professions to participate in the evaluation process. Surprisingly, 21 specialists eventually replied and participated, yielding a 75% response rate. For a study survey of this kind, this response rate is regarded as highly satisfactory and acceptable [39]. The application of judgmental sampling enhances the validity and reliability of the findings and was crucial in guaranteeing that the evaluation comprised only specialists with a thorough understanding of sustainable construction practices [40]. Meanwhile, a list of 21 financial constraints was prepared in accordance with the findings of the literature review and distributed to the experts for careful consideration, analysis, evaluation, and validation. After several rounds of evaluation, the experts came to an agreement on the identification of 12 crucial financial constraints affecting the widespread use of biogenic building materials in the construction industry. The constraints were subsequently coded and arranged as presented in Table 2.
ID | Description |
---|---|
FC1 | Difficulty in upscaling production |
FC2 | Exorbitant regulatory & certification costs |
FC3 | High initial costs of materials |
FC4 | High design & production cost of the materials |
FC5 | Lack of established supply chains |
FC6 | Lack of market standards |
FC7 | Lack of standardization & certification |
FC8 | Limited access to capital and financing options |
FC9 | Limited market availability of biogenic materials |
FC10 | Market prejudice against biogenic resources |
FC11 | Poor funding for research & development |
FC12 | Reluctance to take financial risks |
3.3. Pairwise Comparison of the Financial Constraints using DEMATEL Technique
The experts performed a pairwise investigation of the financial constraints causing the slow adoption of biogenic building materials using the fuzzy DEMATEL technique. In order to determine the influential constraints, this technique was utilized to determine the intricate interactions between the constraints, evaluate the impact of these relationships on one another, and determine the hierarchical relationships between the constraints [41]. Additionally, the tendency of the DEMATEL technique to examine cause-and-effect interactions between system components is its main advantage. To do this, interdependent linkages are transformed into a cause-and-effect group using matrices, and the key problems of complex structural systems are then identified using cause-relation diagrams [42]. As noted by [34], this DEMATEL approach has been extensively employed to describe the intricate relationships among various parts of specific systems particularly in the research fields of construction engineering and management and technology management.
In order to ascertain the constraints’ relative causes, determine the dominant and active constraints, and produce the ranking of the interrelationships among the constraints, the DEMATEL technique was used in this paper. Consequently, during the process of the pairwise investigation of the constraints, the experts were provided with an evaluation scale of zero (0) to three (3) and requested to evaluate the direct influence among any two financial constraints. The values on the evaluation scale were interpreted and explained to the experts as follows: a score of 0 indicates no direct influence, a score of 1 indicates a weak influence, a score of 2 indicates a moderate influence, and a score of 3 indicates a significant influence between the components.
3.4. The Uncertainty Analysis of the Experts’ Pairwise Comparisons
Generally, uncertainty analysis enables the identification of limitations in scientific knowledge and evaluating their implications for scientific conclusions [43]. Therefore, it relevant in this study to ensure that the assessment conclusions provide reliable information for decision‐making [41]. In an expert-based survey of this nature that required the respondents to perform pairwise comparisons of the financial constraints, it is likely that the experts may have different interpretations and perspectives when providing their judgments. In view of this, the fuzzy DEMATEL technique is highly effective in handling these subjective opinions of the experts particularly in the context of analysing financial constraints [43]. The DEMATEL incorporates fuzzy logic, allowing for the representation of imprecise or vague information. This feature enables experts to express their opinions in a nuanced manner, considering the uncertainties and ambiguities inherent in subjective assessments [42]. Thus, the Fuzzy logic helps in accommodating the variability in expert opinions and provides a more flexible basis for analysis. By considering this variability, it becomes much easier to understand the potential impact of differing expert opinions on the analysis [43].
While the DEMATEL requires the experts to provide pairwise comparisons of the relationships between financial constraints, the technique facilitates a systematic approach to capturing and organizing the subjective opinions of experts by structuring these comparisons [41]. Thus, this structured process helps in standardizing and making sense of expert judgments, which enhances the reliability and robustness of the results obtained [41]. In addition, the DEMATEL facilitates the process of building consensus among experts by aggregating their individual opinions into a collective representation of the relationships between financial constraints. Through iterations, experts converged towards a shared understanding of the interconnections within the system. This consensus-building process helps in reducing the impact of individual biases and enhancing the reliability of the results [43]. Not that alone, in DEMATEL, experts assign weights to the pairwise relationships between financial constraints based on their judgments. By quantifying these weights, the technique allows for the prioritization of relationships according to their perceived importance. This weighting mechanism helps in capturing the relative significance of different constraints as perceived by experts, adding a layer of objectivity to the analysis [41].
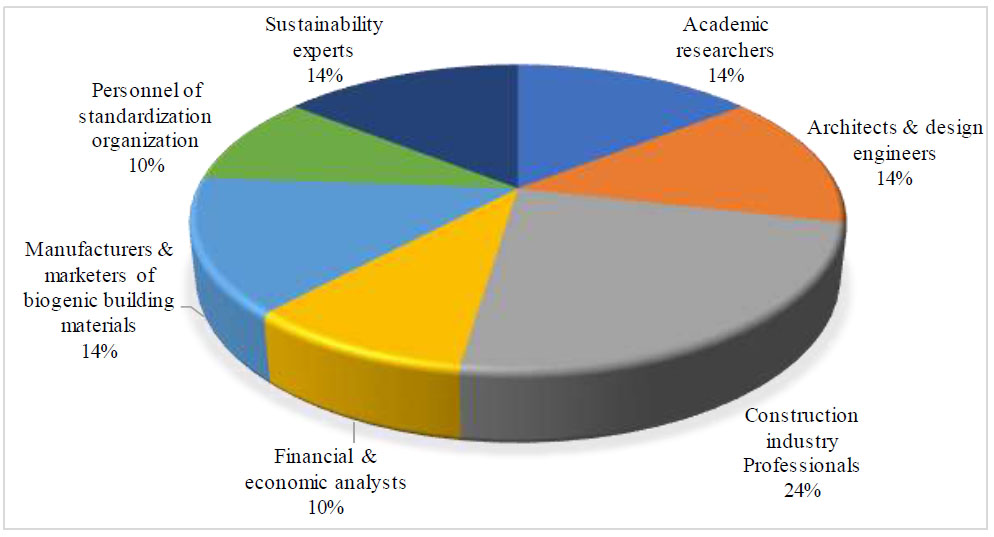
Distribution of the specialists that participated in the expert-based survey.
In essence, the DEMATEL technique effectively handles the subjective opinions of experts by structuring pairwise comparisons, incorporating fuzzy logic, weighting relationships, and facilitating consensus building. These features collectively contribute to mitigating biases, managing uncertainties in expert judgments, and enhancing the reliability and robustness of the results obtained in analysing financial constraints affecting the adoption of biogenic building materials.
4. RESULTS
4.1. Demographic Characteristics of the Respondents
In this sub-section, five questions were addressed to the respondents to obtain information on their respective profiles. Thus, this sub-section identifies the respondents’ professional background, involvement in projects that incorporate sustainable construction practices, experience specifically related to sustainable construction or biogenic materials, level of expertise in biogenic building materials, and level of experience with policies or regulations related to sustainable construction practices. Accordingly, the data analyses found that the highest proportion of the respondents (24%) that that finally participated in the survey and completed the questionnaire were construction industry professionals (Fig. 2).
The distribution of the respondents’ involvement in projects that incorporate sustainable construction practices presented in Fig. (3) shows that nearly 50% of the respondents have extensive experience of involvement in projects that incorporate sustainable construction practices. This indicates that these professionals have a deep understanding of the challenges and opportunities related to sustainable materials, including biogenic building materials.
In essence, their perceptions can provide detailed perspectives on the financial implications of adopting biogenic building materials, including cost considerations, return on investment analyses, and potential financial constraints. Therefore, taking the respondents’ combined experience into consideration can help to provide innovative solutions like establishing some funding schemes specifically for biogenic materials or promoting policies and regulations that encourage their use. Similarly, the distribution of the respondents’ experience specifically related to sustainable construction or biogenic materials shown in Fig. (4) suggests that about 60% of the respondents have a minimum of 8 years of experience in dealing with sustainable construction or biogenic materials.
This indicates that this finding holds important implications for this survey. The respondents experience in sustainable construction or biogenic materials enriches the survey by providing experienced assessments on financial constraints. Their wealth of experience and industry knowledge can inform the survey findings, offering practical solutions and strategic recommendations to facilitate the wider adoption of biogenic building materials within the construction sector. Further, Fig. (5) presents the distribution of the respondents’ level of expertise in biogenic building materials. About 57% of the respondents have advanced level of expertise while 20% are at the level of experts.
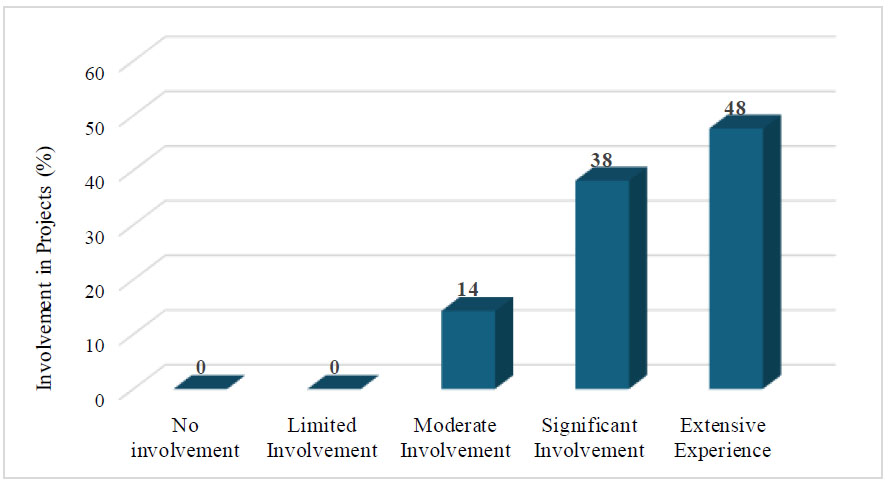
Distribution of the respondents’ involvement in projects that incorporate sustainable construction practices.
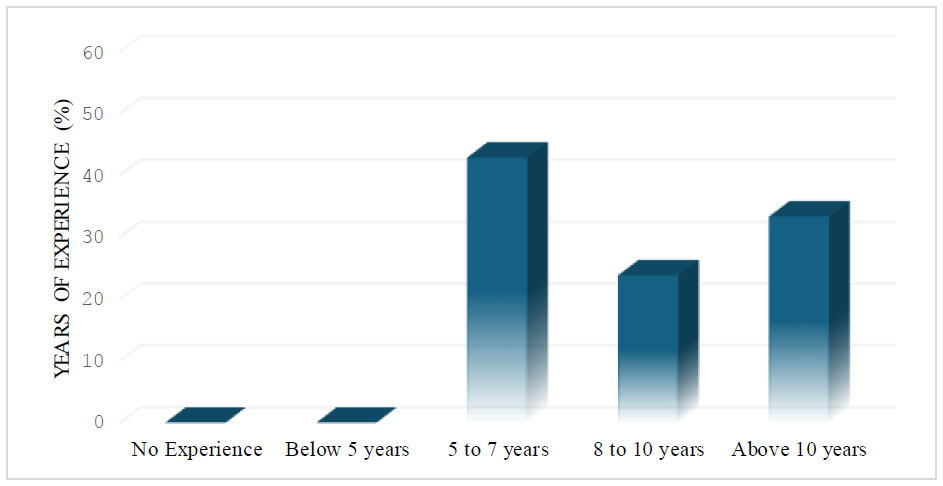
Distribution of the respondents’ experience specifically related to sustainable construction or biogenic materials.
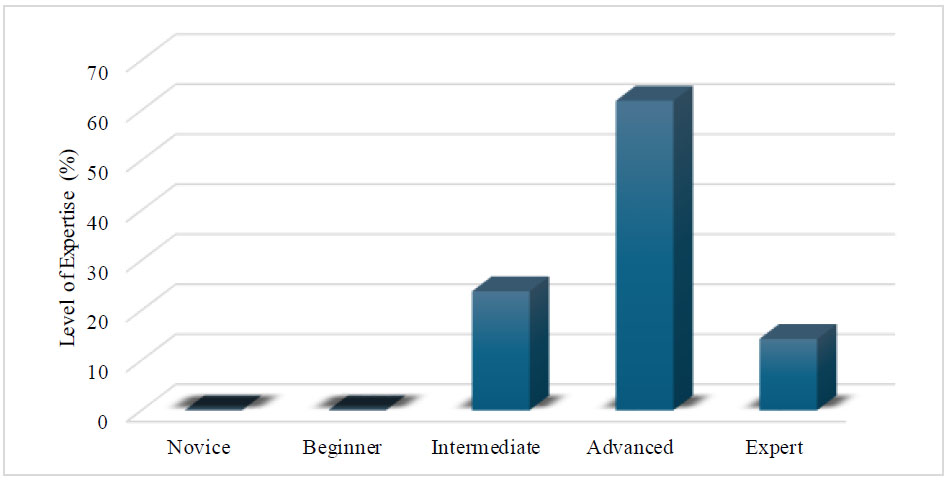
Distribution of the respondents’ level of expertise in biogenic building materials.
This means that their advanced understanding suggests a deep familiarity with the technical aspects, benefits, and challenges of utilizing biogenic materials in construction projects. Their judgments can contribute to informed decision-making processes that consider the financial implications of adopting biogenic materials, balancing cost considerations with environmental benefits.
Meanwhile, the distribution of the respondents’ level of experience with policies or regulations related to sustainable construction practices is shown in Fig. (6). It can be deduced from the figure that nearly 30% of the respondents have moderate experience with policies or regulations related to sustainable construction practices, while about 50% have extensive experience and about 10% are at the level of policy development. Their experience indicates a familiarity with regulatory frameworks that may impact the adoption of biogenic building materials, including standards, incentives, and reporting requirements. In addition, their understanding can provide insights into how regulatory requirements may influence the cost considerations and financial feasibility of incorporating biogenic materials in construction projects. In general, their knowledge can help guide the development of strategies to overcome financial constraints in sustainable construction projects and provide a better understanding of how policy frameworks affect the financial considerations surrounding the adoption of biogenic building materials.
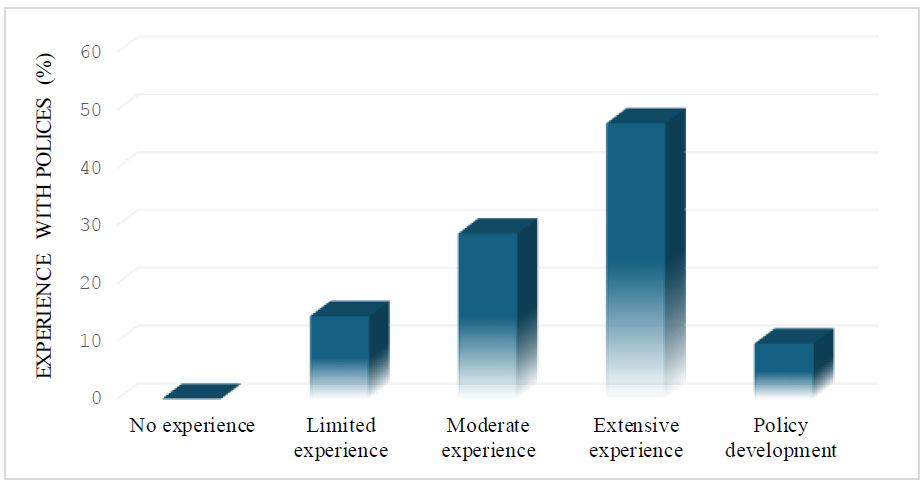
Distribution of the respondents’ level of experience with policies or regulations related to sustainable construction practices.
Constraints | FC12 | FC11 | FC10 | FC9 | FC8 | FC7 | FC6 | FC5 | FC4 | FC3 | FC2 | FC1 | Total |
---|---|---|---|---|---|---|---|---|---|---|---|---|---|
FC1 | 2 | 2 | 2 | 3 | 0 | 1 | 1 | 2 | 2 | 0 | 0 | 1 | 12 |
FC2 | 0 | 3 | 0 | 3 | 0 | 3 | 0 | 3 | 0 | 0 | 1 | 0 | 10 |
FC3 | 3 | 3 | 3 | 3 | 3 | 2 | 3 | 3 | 3 | 1 | 2 | 3 | 26 |
FC4 | 3 | 3 | 3 | 3 | 0 | 1 | 3 | 3 | 1 | 3 | 3 | 0 | 20 |
FC5 | 0 | 0 | 0 | 0 | 0 | 0 | 0 | 1 | 0 | 0 | 1 | 2 | 4 |
FC6 | 3 | 3 | 3 | 0 | 1 | 1 | 1 | 2 | 0 | 0 | 2 | 0 | 10 |
FC7 | 3 | 2 | 3 | 0 | 2 | 1 | 0 | 2 | 3 | 3 | 1 | 3 | 18 |
FC8 | 3 | 3 | 3 | 0 | 1 | 3 | 3 | 0 | 0 | 0 | 0 | 0 | 10 |
FC9 | 2 | 2 | 2 | 1 | 2 | 1 | 2 | 2 | 0 | 0 | 1 | 3 | 14 |
FC10 | 1 | 1 | 1 | 0 | 0 | 2 | 0 | 0 | 0 | 0 | 0 | 0 | 3 |
FC11 | 0 | 1 | 1 | 0 | 0 | 0 | 0 | 0 | 0 | 0 | 0 | 0 | 1 |
FC12 | 1 | 0 | 1 | 0 | 0 | 0 | 0 | 0 | 0 | 0 | 2 | 0 | 3 |
4.2. Results of the Pairwise Comparison of the Financial Constraints
Through expert judgment explained before now, the “direct influence matrix” displayed in Table 3 was created to indicate relative interconnectedness among the identified constraints. Not only that, but it was also necessary to identify the most important constraints and the order in which they should be interrelated. The “direct influence matrix” thus shows the direct and indirect effects of all the constraints. A threshold value of 0.10 was established by taking the average of all the constraints in the whole identity matrix.
The experts were requested to assess the direct influence among any two constraints with an integer value ranging from 0 to 3, with 0, 1, 2, and 3 representing no, low, medium and high influence respectively. Thus, mathematically, a n x n non-negative matrix was created for each respondent as (Eq. 1).
![]() |
(1) |
Where k is the number of respondents with 1 ≤ k ≤H (with H being the total number of respondents) and n is the total number of financial constraints adopted in the study. Both i and j rang from 1 to n, and define each element of n x n response matrix. This will yield H response matrices (X1, X2, ... ... . XH). To integrate the response of all H respondents, an n x n average direct influence matrix A=[aij], shown in Table 3, was obtained as (Eq. 2).
![]() |
(2) |
The “direct influence matrix (Table 3)” was the first step in determining the degree of influence displayed in Tables 4-6, followed by the “normalized direct initial matrix (Table 4)” and the “total identity matrix (Table 5)”. Based on the values obtained in Table 6 (degree of influence), the causal relationship diagram was established (Fig. 7). From Table 6, it can be seen that the “D”, “R”, “D+R” and “D-R” numbers for each financial constraint have been provided. Usually, the “D” represents the sum of each row of the total identity matrix, which is the extent to which each constraint is influenced by other constraint while the “R” represents the sum of each column of the total identity matrix, which is the extent to which each constraint directly or indirectly affects other constraints. Thus, mathematically, the normalized direct initial matrix (D) shown in Table 4 is determined as (Eq. 3);
![]() |
(3) |
- | FC12 | FC11 | FC10 | FC9 | FC8 | FC7 | FC6 | FC5 | FC4 | FC3 | FC2 | FC1 |
---|---|---|---|---|---|---|---|---|---|---|---|---|
FC1 | 0.077 | 0.077 | 0.077 | 0.115 | 0.000 | 0.038 | 0.038 | 0.077 | 0.077 | 0.000 | 0.000 | 0.038 |
FC2 | 0.000 | 0.115 | 0.000 | 0.115 | 0.000 | 0.115 | 0.000 | 0.115 | 0.000 | 0.000 | 0.038 | 0.000 |
FC3 | 0.115 | 0.115 | 0.115 | 0.115 | 0.115 | 0.077 | 0.115 | 0.115 | 0.115 | 0.038 | 0.077 | 0.115 |
FC4 | 0.115 | 0.115 | 0.115 | 0.115 | 0.000 | 0.038 | 0.115 | 0.115 | 0.038 | 0.115 | 0.115 | 0.000 |
FC5 | 0.000 | 0.000 | 0.000 | 0.000 | 0.000 | 0.000 | 0.000 | 0.038 | 0.000 | 0.000 | 0.038 | 0.077 |
FC6 | 0.115 | 0.115 | 0.115 | 0.000 | 0.038 | 0.038 | 0.038 | 0.077 | 0.000 | 0.000 | 0.077 | 0.000 |
FC7 | 0.115 | 0.077 | 0.115 | 0.000 | 0.077 | 0.038 | 0.000 | 0.077 | 0.115 | 0.115 | 0.038 | 0.115 |
FC8 | 0.115 | 0.115 | 0.115 | 0.000 | 0.038 | 0.115 | 0.115 | 0.000 | 0.000 | 0.000 | 0.000 | 0.000 |
FC9 | 0.077 | 0.077 | 0.077 | 0.038 | 0.077 | 0.038 | 0.077 | 0.077 | 0.000 | 0.000 | 0.038 | 0.115 |
FC10 | 0.038 | 0.038 | 0.038 | 0.000 | 0.000 | 0.077 | 0.000 | 0.000 | 0.000 | 0.000 | 0.000 | 0.000 |
FC11 | 0.000 | 0.038 | 0.038 | 0.000 | 0.000 | 0.000 | 0.000 | 0.000 | 0.000 | 0.000 | 0.000 | 0.000 |
FC12 | 0.038 | 0.000 | 0.038 | 0.000 | 0.000 | 0.000 | 0.000 | 0.000 | 0.000 | 0.000 | 0.077 | 0.000 |
- | FC12 | FC11 | FC10 | FC9 | FC8 | FC7 | FC6 | FC5 | FC4 | FC3 | FC2 | FC1 |
---|---|---|---|---|---|---|---|---|---|---|---|---|
FC1 | 0.220 | 0.241 | 0.223 | 0.226 | 0.061 | 0.145 | 0.140 | 0.209 | 0.144 | 0.051 | 0.085 | 0.110 |
FC2 | 0.105 | 0.248 | 0.109 | 0.192 | 0.037 | 0.203 | 0.074 | 0.212 | 0.036 | 0.035 | 0.102 | 0.032 |
FC3 | 0.341 | 0.373 | 0.349 | 0.279 | 0.215 | 0.245 | 0.265 | 0.322 | 0.223 | 0.115 | 0.215 | 0.242 |
FC4 | 0.315 | 0.351 | 0.324 | 0.265 | 0.086 | 0.197 | 0.248 | 0.296 | 0.139 | 0.187 | 0.225 | 0.101 |
FC5 | 0.015 | 0.015 | 0.017 | 0.006 | 0.005 | 0.010 | 0.009 | 0.046 | 0.004 | 0.002 | 0.049 | 0.081 |
FC6 | 0.232 | 0.261 | 0.238 | 0.098 | 0.092 | 0.136 | 0.118 | 0.192 | 0.063 | 0.034 | 0.142 | 0.064 |
FC7 | 0.257 | 0.240 | 0.262 | 0.107 | 0.142 | 0.152 | 0.096 | 0.205 | 0.181 | 0.149 | 0.123 | 0.198 |
FC8 | 0.249 | 0.273 | 0.252 | 0.104 | 0.102 | 0.217 | 0.196 | 0.133 | 0.075 | 0.044 | 0.079 | 0.078 |
FC9 | 0.197 | 0.213 | 0.201 | 0.126 | 0.129 | 0.129 | 0.153 | 0.186 | 0.061 | 0.040 | 0.114 | 0.181 |
FC10 | 0.082 | 0.092 | 0.083 | 0.034 | 0.019 | 0.110 | 0.027 | 0.043 | 0.020 | 0.010 | 0.026 | 0.020 |
FC11 | 0.017 | 0.062 | 0.056 | 0.018 | 0.010 | 0.017 | 0.013 | 0.021 | 0.010 | 0.006 | 0.012 | 0.011 |
FC12 | 0.061 | 0.028 | 0.065 | 0.021 | 0.011 | 0.016 | 0.017 | 0.022 | 0.015 | 0.007 | 0.089 | 0.014 |
ID | Description of Financial Constraints | Row Total (D) | Column Total (R) | (D+R) Values | (D-R) Values |
---|---|---|---|---|---|
FC1 | Difficulty in upscaling production | 1.39 | 1.132 | 2.53 | 0.26 |
FC2 | Exorbitant regulatory & certification costs | 1.03 | 1.263 | 2.30 | -0.23 |
FC3 | High initial costs of materials | 2.47 | 0.680 | 3.15 | 1.79 |
FC4 | High design & production cost of the materials | 2.07 | 0.971 | 3.04 | 1.10 |
FC5 | Lack of established supply chains | 0.23 | 1.887 | 2.12 | -1.66 |
FC6 | Lack of market standards | 1.18 | 1.355 | 2.53 | -0.18 |
FC7 | Lack of standardization & certification | 1.62 | 1.245 | 2.86 | 0.37 |
FC8 | Limited access to capital and financing options | 1.28 | 0.910 | 2.19 | 0.37 |
FC9 | Limited market availability of biogenic materials | 1.32 | 1.477 | 2.80 | -0.16 |
FC10 | Market prejudice against biogenic resources | 0.39 | 2.178 | 2.57 | -1.78 |
FC11 | Poor funding for research & development | 0.17 | 2.397 | 2.57 | -2.22 |
FC12 | Reluctance to take financial risks | 0.28 | 2.091 | 2.37 | -1.81 |
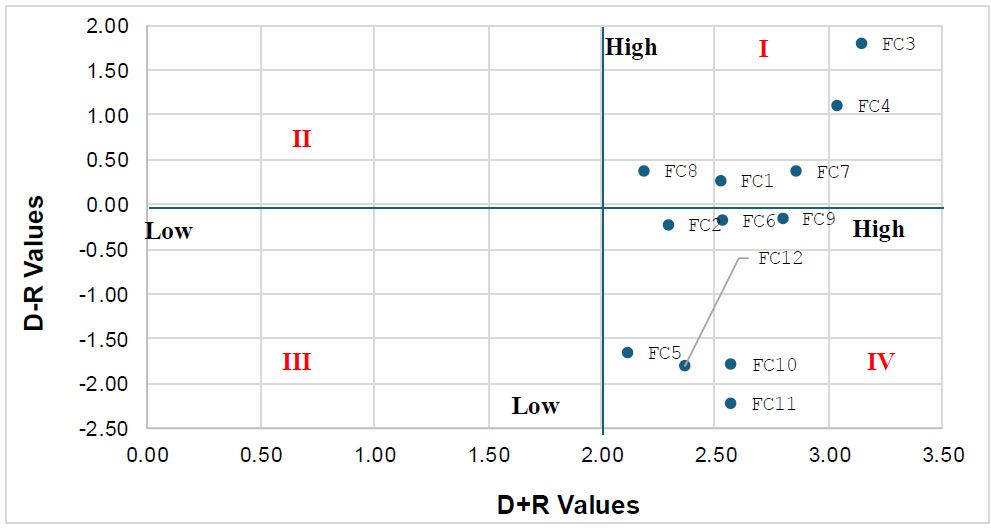
The causal relationship diagram.
The total identity matrix (T) shown in Table 5 was obtained through Eq. (4) below.
![]() |
(4) |
Where I is the identity matrix
The sum of rows (D) and columns (R) of the total identity matrix (T) were obtained as:
![]() |
(5) |
![]() |
(6) |
In Eqs. (5 and 6), tij represents each element of the total identity matrix shown in Table 5 and Eq. (4).
The sum of columns represents the direct and indirect effect of factor i on the other constraints while the sum of rows represents the direct and indirect effects the constraint j receives from the other constraints. Additionally, the sum (Di + Ri) shows the total effects given and received by constraint i, whereas the difference (Di-Ri) indicates the net effects that constraint i contributes to the system. In other words, if (Di-Ri) is positive, constraint i is a net cause, while if (Di-Ri) is negative, constraint i is a net receiver. Accordingly, the sum (Di+Ri) and the difference (Di-Ri) are arranged in the degree of influence shown in Table 6 and are graphically mapped (Fig. 7) to rationally visualize and interpret the complex relationship among all the constraints.
Further, the “D+R” stands for centrality, which represents the strength of relationship between the constraints. For instance, if the value of the “D+R” is closer to the right of the causal diagram (Fig. 7), this indicates high strength of the relationship and low strength (weakness) if closer to the left. On the other hand, the “D-R” stands for causality, which represents significance of the constraint in terms of strength of influence. The constraints are divided into net causers and net receivers based on the “D-R” values of the constraints. If a constraint's “D-R” value is positive, it is regarded as a net causer; if it is negative, it is seen as a net receiver. Accordingly, the higher negative “D-R” values indicate a poorer level of influence, while the higher positive “D-R” values generally indicate a stronger level of cause.
A closer look at Fig. (7) will show that the causal diagram has four distinct quadrants; each quadrant is represented by a numerical number (I, II, III, and IV). To begin with, constraints that fall into the first quadrant (I) tend to have positive values of “D – R” and very high values of “D + R”. These constraints are regarded as the critical driving constraints to solve the core problems and thus should be the priorities for treatment. Any action taken on these type of constraints has wide range impact on the other constraints. Similarly, constraints in the second quadrant (II) tend to have positive values of “D – R” and very small values of “D + R”. These constraints are considered as slightly independent and influential but cannot be easily influenced by other constraints. Further, constraints in the third quadrant (III) tend to have negative values of “D – R” and very small values of “D + R”. These constraints affect other constraints and are also affected by the other constraints. Meanwhile, constraints in the fourth quadrant (IV) have negative values of “D – R” and large values of “D + R”. Constraints in this quadrant are affected by other constraints and require more attention though they are not urgent priority to be dealt with.
4.3. Results Validation
For the results of this study to be considered reliable, accurate, and applicable to actual situations in the construction sector, validation is essential. By adopting the expert validation approach, the feedback of the diverse experts that participated in the expert-based survey (Fig. 2) was sought in order to cross-validate the results obtained through the DEMATEL analysis. The results of the DEMATEL analysis were presented to the experts and their satisfactory feedback on the identified constraints and relationships was gathered as it offered practical insights and validation of the relevance of the results in the industry. This process helped in confirming the identified influential constraints, intricate interactions, and hierarchical relationships between the financial constraints. To further enhance the credibility and reliability of the results obtained from the study, peer review of the results was also performed, which helped to provide an independent evaluation of the research methodology, analysis techniques, and results obtained through the DEMATEL approach. Accordingly, the validity of the study was strengthened by including the feedback and suggestions obtained from the peer review approach.
5. DISCUSSION
Prior to the commencement of this study, there was published journal or conference article that explicitly applied the DEMATEL modelling technique to define the intricate relationships between the financial constraints to the adoption of biogenic building materials, evaluate the effects of these relationships, ascertain the influential barriers, and determine the hierarchical relationships between the constraints. Therefore, the objective of this study is to provide a deeper examination and understanding of these financial constraints in order to propose strategies to overcome them, rather than directly criticizing current knowledge. By doing this, it will be possible to put in place effective policies that will accelerate the construction industry's widespread adoption of biogenic building materials.
Going by the results obtained in the previous section, it is obvious that the most significant and influential financial constraints are high initial costs of materials (FC3), high design & production cost of the materials (FC4), lack of standardization & certification (FC7), difficulty in upscaling production (FC1), and limited access to capital and financing options (FC8). Coincidentally, these constraints fall under the first quadrant in Fig. (7) and are thus, regarded as the critical driving constraints that should be given due attention to promote the wide adoption of biogenic building materials in the construction sector. In essence, the results of this study are consistent with other research highlighting some of the critical constraints to the adoption of green building materials in construction.
The high initial costs of biogenic building materials is the most critical constraint to the adoption of biogenic materials in building construction [9]. This is because building with biogenic materials is not only about using a different product, but also requires an entirely different approach to design, technology development, and innovative construction cost models [1]. All of this generates a major reluctance to make the upfront investment in time, labour, materials, and resources. If a developer does decide to build with bio-based materials, the learning costs can be significant as both the cost and the risk are considerable. Similarly, the higher design and production costs associated with biogenic building materials is considered a critical financial barrier in their adoption because designing with biogenic materials requires uncommon expertise, leading to higher design costs [10]. Also, adapting biogenic materials into existing systems may require modifications, increasing design complexity and costs while rigorous quality assurance processes and compliance requirements add to the design expenses [11].
Not that alone, risk assessment for novel biogenic materials demands additional resources and increases design costs [9]. Other aspects that lead to higher design cost of biogenic materials include prototyping and testing for performance evaluation which can be time-consuming and costly as well as scalability and standardization, where limited production scale contributes to higher design costs due to customization requirements and lack of standardized solutions [2]. Not that alone, high production costs are cited as among the primary factors discouraging the increase in the use of biogenic materials in mass building projects [1]. The complexity, as well as the need for carefully trained technicians and workers in the transformation processes, also represents a significant portion of the expenditures for the transformation of biomasses into construction products [23]. Thus, the use of biogenic materials is ultimately hampered by these issues, which increase upfront costs, necessitate specialist knowledge, and make the design process more difficult.
Another crucial financial constraint is the lack of standardization and certification. The significance of standardization and certification lies in its ability to mitigate risks and minimize expenses [25]. The entire construction industry is based on the supply of certified materials, as the manufacture and construction of buildings would be considerably complicated without adhering to the required standards for material safety, performance, or technological function [25]. Lack of standardization or non-compliance with construction requirements does not allow successful market entry for any construction material. Thus, biogenic building materials require consistent, high-quality standards and certifications to overcome variability and market acceptance issues [29]. Standardization reduces risks associated with new materials, such as fire resistance and structural integrity [9]. Lack of certifications can discourage developers and investors from adopting biogenic materials, compromising their economic viability [23]. Accredited certifications can distinguish biogenic products by emphasizing their sustainability and environmental advantages, potentially increasing demand, reducing costs, and opening up new opportunities in construction projects.
Difficulty in upscaling production is also one of the top-rated financial constraints to the adoption of biogenic building materials [2]. Upscaling production is crucial for achieving economies of scale in biogenic materials, as it reduces unit costs and increases market demand. A well-optimized supply chain is essential for scalability, as inefficiencies can lead to increased costs and disruptions. While difficulty in upscaling production can hinder access to financing, putting biogenic material suppliers at a disadvantage, limited production capacity also limits the market penetration and growth potential of biogenic materials. This absolutely limits their overall impact and adoption in the construction industry [2].
Limited access to capital and financing options also presents a critical financial barrier to the adoption of biogenic building materials in the construction industry because biogenic materials often have higher initial costs compared to traditional construction materials [1]. In view of this, limited access to capital makes it quite difficult for developers and builders to afford the upfront expenses associated with purchasing and incorporating these building materials into their construction projects [11]. For this reason, this financial barrier can discourage investment in biogenic materials, hindering their adoption in the construction sector [9]. Biogenic materials may be perceived as riskier investments due to their novelty and unproven long-term performance [10]. This can lead to limited access to capital for projects involving untested materials. Additionally, biogenic materials may lack established collateral value, making securing loans challenging [23]. Financial institutions and investors may also have limited knowledge about biogenic materials and their benefits. Additionally, uncertainties related to building codes and regulations can further complicate securing funding for biogenic materials [29]. On the whole, overcoming the challenge of limited access to capital and financing options is vital for promoting the wider adoption of biogenic building materials in the construction industry [9]. This could be achieved by providing the necessary financial support for projects incorporating these sustainable materials.
5.1. Strategies for Overcoming the Financial Constraints
Public policy and financial assistance are crucial in deciding the use of biogenic building materials. These can include tax credits, grants, low-interest loans, and subsidies. Expanding funding sources, such as green financing models and collaborations with sustainable construction institutions and investors, can help set up investments and provide access to funding, favourable terms, and financial expertise. Nonetheless, it is important to develop financial models that will consider the long-term costs and benefits of using biogenic building materials, considering factors like raw materials, technology, production, logistics, construction, main- tenance, insurance, market risks, and bank interests. Risk aversion and transparency are crucial. Trustworthy software and financial assistance can overcome market barriers and expedite the use of specific biogenic building materials in all projects.
On the other hand, industry stakeholders can develop harmonized standards to evaluate impacts and enhance awareness. For instance, professionals can benefit from dedicated training programs and workshops. While a common certification system can address branding challenges and promote collaboration, technological advancements can support the development of a new certification tool. Other notable strategies to overcome these financial challenges include promoting awareness of the benefits and improvements to overcome the perceived challenges and help to enhance market acceptance associated with it. Not that alone, growing consumer awareness and demand for sustainable construction practices can create market opportunities for projects using biogenic materials, enhancing competitiveness and profitability. Despite financial constraints, the construction industry requires efficient, high-quality, and timely supply chains for biogenic building materials to navigate regulatory and stock-related volatility. Thus, developing market standards for biogenic building materials can promote market acceptance, reduce costs, and foster innovation in the construction industry. This strategy can enhance efficiency, boosts competitiveness, and builds confidence by reducing investment risks and increasing credibility.
CONCLUSION
The study discussed in this paper looked at the key financial constraints to the adoption of biogenic building materials. The findings point to five major constraints that have the biggest influence on other financial constraints and are crucial in influencing the wide adoption of biogenic building materials in the construction industry. Essentially, the findings indicate that the most highly prioritized constraints with the strongest level of cause on the other financial constraints are high initial costs of materials (FC3), high design & production cost of the materials (FC4), lack of standardization & certification (FC7), difficulty in upscaling production (FC1), and limited access to capital and financing options (FC8). This study contributes to advancing knowledge in sustainable construction practices by determining and understanding the specific financial constraints that impede the widespread adoption of biogenic building materials within the construction industry. Additionally, the study offers practical solutions for industry stakeholders and has the potential to influence policies aimed at promoting environmentally friendly building materials. By addressing the financial constraints to adopting biogenic materials, the study promotes sustainable practices within the construction sector, contributing to environmental conservation and resource efficiency.
Despite the study's contributions, there are some shortcomings related to the study. It is important to bear in mind when evaluating the findings reported in this paper that the study was restricted to the United Arab Emirates and Saudi Arabia. This will help to apply caution in case of projecting the results to other Gulf nations. Similar to that, the study's findings may not be as broadly applicable as they may be because of the small sample size on which they were based. Nonetheless, the findings were further supported by the fact that the experts' judgment was regarded as extremely reliable and this further increased the validity of the findings. As a result, it is hereby confirmed that all the financial constraints examined in this paper are essentially the views of academic researchers, architects & design engineers, construction industry professionals, financial & economic analysts, manufacturers & marketers of biogenic building materials, personnel of standardization organization, and sustainability experts in the United Arab Emirates and Saudi Arabia. Notwithstanding the limitations of the study mentioned above, this study provides essential knowledge and evidence that might be used to promote the widespread use of biogenic building materials in the construction industry.
Essentially, the major managerial implication of this study is that managers can use the study's findings to develop strategic plans that incorporate the use of biogenic building materials, considering both the financial implications and the long-term benefits for their projects. Also,
engagement with relevant stakeholders in the industry can foster collaboration and help in overcoming resistance to adopting new materials and practices. As for the practical implications, the study findings could be useful in reducing the environmental footprint of construction projects thereby contributing to sustainability goals. Not that alone, construction companies that adopt biogenic materials following the study's recommendations may enjoy cost savings in the long-term and gain a competitive edge by aligning with the growing demand for sustainable construction practices. Overall, the study's managerial and practical implications are extensive and have the potential to enhance decision-making, operations, and sustainability initiatives within the construction sector.
Meanwhile, the study opens up various avenues for future research and practical applications. Thus, based on the findings and objectives of the study, future research could explore deeper into conducting cost-benefit analyses of adopting biogenic building materials. This analysis could consider the long-term economic benefits, environmental impacts, and social implications associated with the widespread adoption of such materials in construction projects. Not that alone, the future research could be conducted to investigate emerging technologies that could enhance the affordability, durability, and performance of biogenic building materials, thereby reducing financial constraints and increasing their adoption rate.Most importantly, the future research could undertake case studies to showcase successful implementations of biogenic building materials in real-world projects. Analyze the challenges faced, lessons learned, and best practices that can be applied to future construction projects aiming for zero carbon buildings.
AUTHORS' CONTRIBUTION
The author confirms sole responsibility for the following: study conception and design, data collection, analysis and interpretation of results, and manuscript preparation.
LIST OF ABBREVIATIONS
FC | = Financial Constraints |
DEMATEL | = Decision-making Trial And Evaluation Laboratory |