All published articles of this journal are available on ScienceDirect.
Energy Performance of Dwellings in a Temperate Climate Area of Argentina. An Architectural Proposal
Abstract
Aim:
The research aimed at carrying out a global comparative synthesis of the results of monitoring thermal energy behavior of single-family dwellings and apartments located in the city of Santa Rosa, La Pampa, Argentina; and describing an architectural proposal that considers use of resources (land, morphology, materials and energy), lifestyle of inhabitants (qualitative variables) and critical analysis of the historical problems of the housing deficit in Argentina.
Methods:
The work includes a typological analysis of clusters of dimensional, morphological and thermo-physical indicators, energy performance and inhabitant’s behavior. Regarding energy heating consumption, multifamily buildings consume 52% less energy than the average single-family dwellings.
Results:
An architectural proposal taken into account the use of resources and energy efficiency strategies to give an answer to the housing deficit of the area under study showed that the densification of housing provides significant energy and economic benefits, especially if one incorporates passive solar design principles. Apartment blocks (B) and single-family dwellings (H) show 114.3 and 47.6 dwelling/hectare, and as a result, 39.4 and 16.4 inhabitant/hectare.
Conclusion:
Heating energy saving of B in relation to H is around 32% and the envelope’s cost is reduced to 47%. Argentina’s energy scenario, the possibility of reviewing regulations and building codes, the region’s growth trend in housing construction, the process of building labelling, among others, require a thorough analysis of the information on characteristics of building stock and its energy performance.
1. INTRODUCTION
Buildings provide a living and working environment to human beings. Eighty percent of living activities of people take place inside buildings, therefore, special attention should be given to both the quality of indoor environments during the design phase and the evaluation of their energy-environmental performance [1]. Buckminster Fuller’s premonitory vision about the finiteness of the world’s resources goes back to his final notes of 1920. It was was not until 1973, with the high oil prices, that energy conservation strategies were set on the environmental agenda. Actually, it calculates The Earth Overshoot Day [2]. This year on July 29, humanity will have used nature’s resource budget for the entire year, according to Global Footprint Network, an international sustainability organization that has pioneered the Ecological Footprint, the earliest ever. The construction industry has a significant impact on the environment. Hence, while buildings provide facilities for human needs and their countless benefits to the society cannot be ignored, buildings have also had destructive influences on the environment during the last decades [3]. According to some studies, construction is responsible for upto 50% of climate change, 40% of energy usage globally, and 50% of landfill waste, not to mention air, water, and noise pollution and destruction of natural habitats. IPCC [4] considers that design strategies for energy-efficient buildings must include a reduction of heating and cooling loads, a selection of systems allowing a more effective use of energy resources, an efficient use of equipment and the effective control of conditioning strategies. An improvement in energy performance requires a detailed study and simulation by modelling thermo-energy behavior, while considering the phenomena taking place, for example, on an urban scale. They state that building energy performance depends on urban geometry, building design, system efficiency and, most importantly, inhabitant’s behavior. Dixit et al. claimed that there is an urgent need for a review and modification of current construction practices in terms of design, techniques and manufacturing technologies in order to optimize energy consumption [5]. Liu et al. maintained that in order to create low-energy consumption buildings, the development of new materials, technologies and systems, in addition to knowledge, is needed [1].
Regarding the energy situation in Argentina, the Argentinean Ministry of Energy and Mining [6] asserts that Natural Gas (NG) is the main component of the national energy matrix, with the contribution of more than 50% of the country's primary energy; about 27% of the gas is distributed to residential users through networks. Residential consumption has had a rapid growth in recent years. In 1990, this consumption represented 21% of the total, while in 2015 its participation reached 27% [7]. Specifically, in residential consumption, it is important to note that Natural Gas (NG) consumption increased five times than that of electricity, 13.4 and 3 MWh/year, respectively. Gastiarena et al. affirmed that 92% of total Argentinean population has the following scheme of residential NG consumption: 56% corresponds to heating and 34% to water heating (including passive factor) [7]. When comparing specific residential consumption, both electric and NG consumption, at the Argentinean level, the specific consumption of NG by networks is a factor 4 greater than the average residential electrical consumption. Both could be reduced through bioclimatic architectural designs. In the building sector, the in force 2010 census statistics [8] showed that 16.8% of the residents live in apartments and 78.9% in houses with a rate of 3.3 people per dwelling, nevertheless, only 61.6% of the total dwelling in Argentina is habitable, 34.2% needs repair and/or improve basics habitability conditions. Finally, 4.2% should be demolished and rebuilt, since they cannot be restored. Specifically, in the area of study, energy balance shows that the province of La Pampa buys approximately 80% of the energy consumed. In the city of Santa Rosa, the capital of the province, the residential electricity consumption represents 87.2% of the total delivered and, according to a study [9, 10] the energy consumed per user increased by 17% during 2008-2017(2008 = 2134 kWh/user and 2017 = 2576 kWh/user). NG represents a significant portion of the total energy consumption (90%), most of it is being used for heating (67%). Regarding the state of conservation of housing, only 22.1% needs repair and 0.6% rebuilding.
Wright [11], claimed that the buildings energy consumption results from a complex interaction between its design, its location, the energy-using equipment it contains, its occupants and the affordability of fuel. Peeters et al. [12], considered that in residential buildings the internal conditions are far from reaching a state of balance since both the level of activity and the type of clothing can constantly vary, causing fluctuation in energy input which can rapidly affect indoor temperature. Variation in occupation will affect, among others, the ventilation rate required to maintain indoor air quality. Energy efficiency, however, depends on these technologies, on the user’s actions and on the policies influencing decision-making [13].
In this context, the research/study aimed to carry out a global comparative synthesis of the results of monitoring thermal energy behavior of 13 single-family dwellings and apartments located in the city of Santa Rosa, La Pampa, Argentina; and to describe an architectural proposal that considers to use resources (land, morphology, materials and energy), lifestyle of inhabitants (qualitative variables) and critical analysis of the historical problems of the housing deficit in Argentina.
2. MATERIALS AND METHODS
Fig. (1) shows the geographical location of Santa Rosa city (36° 27'S, 64° 27'W, and 189 meters above sea level), capital of La Pampa province. Note that all the national IRAM Standards used in this work are in force despite the fact that the last revisions of someone are old. It belongs to the IIIa Bioenvironmental region [14]. It has a cold-temperate climate with minimum temperature reaching -10°C. The highest maximum temperatures (around 36.4ºC) are registered in January [15]. Fig. (2) shows the town’s most important climatic variables.
Fig. (3) shows the psychometric chart of monthly temperature and humidity average values, mean minimum temperature and maximum relative humidity values, and mean maximum temperature and minimum relative humidity values. The diagram was developed using the software Weather Tool [16].
Only the points corresponding to the maximum average temperature and minimum average relative humidity of April and October were found to be in the comfort zone. For other months, the architectural design considered a series of recommendations to achieve thermal well-being. The neutrality temperature (Tn) was calculated from two models: ASHRAE 55-17 model [17] (for mean outdoor temperatures falling between 10°C and 33.5°C) and the model of Pérez-Fargallo et al. [18] (for mean outdoor temperatures (Tm) 6.5°C to 10°C).
Temperature of thermal neutrality (Tn = 17.8°C + 0.31 Tm for 10°C < Tm < 33.5 °C; Tn =13.6°C + 0.678 Tm for 6.5°C < Tm < 10°C) and adaptive comfort zone for July and January (thermal acceptability of 90%) are 19.4 and 25.2ºC for July and January, respectively. Towards the right of the comfort zone, the most highly vulnerable situation corresponds to the maximum average temperature values and minimum average relative humidity values evidenced in January, during which mechanical ventilation is needed in 62.5% of the day. To the left of the graph, for mean minimum temperature values and maximum average relative humidity values in July, auxiliary heating is required during the 24 hours of the day to be in a comfort zone. The minimum winter design temperature is -6ºC according to a study [14]. The IRAM Standard 11605:2004 [19] recommends 0.30, 0.80 and 1.39W/m2ºC (Levels A, B and C) for walls, and 0.26, 0.67 and 1.00 W/m2ºC for roofs.
Fig. (4) shows the location of the cases studied. It details the plants and views of dwellings without thermal insulation in their envelope. It should be noted that none of the houses reach the minimum level as suggested in a study [19] that specifies maximum U-values required for walls and roofs, according to the country’s different bioenvironmental regions.
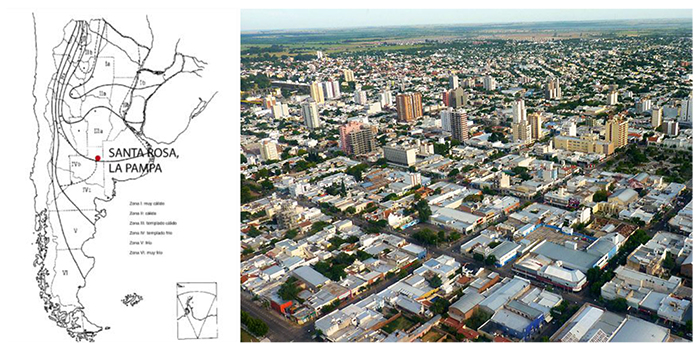
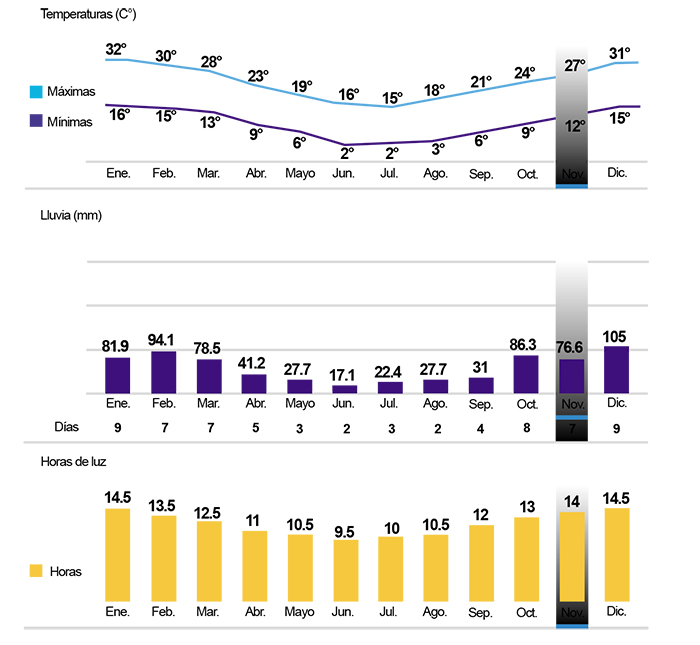
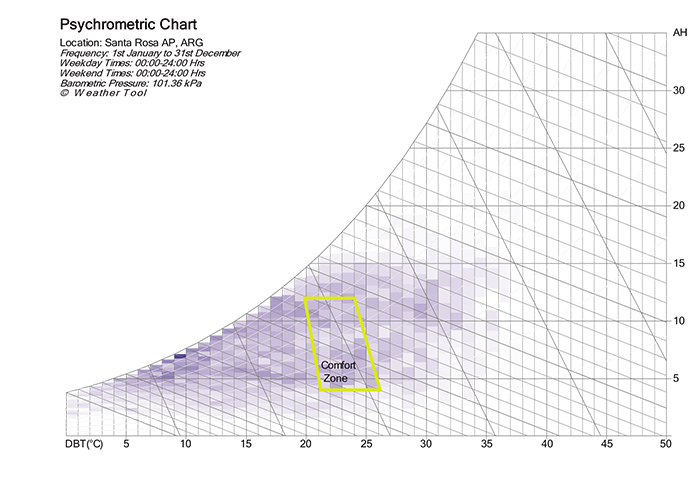
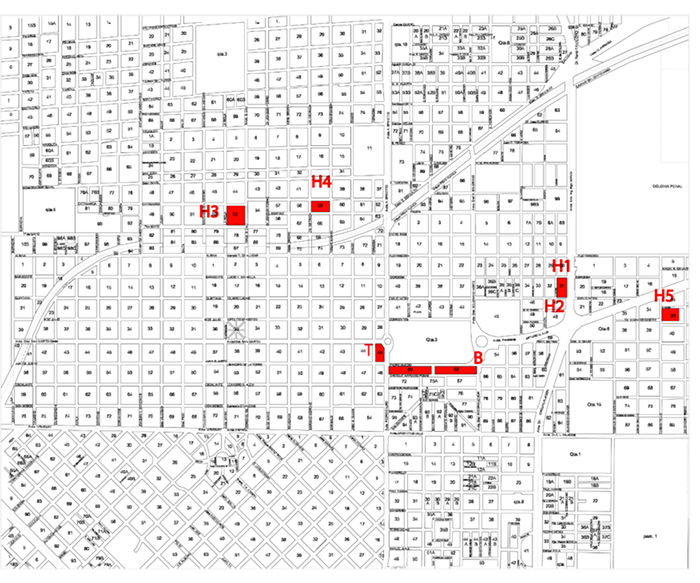
The case studies comprise: Single-family Dwellings (H); Apartments in Block Buildings (B); and Apartments in Tower Building (T). H refers to one-floor houses with private backyard built with high thermal inertia materials (400Kg/m2). H1 to H4 are located in low-density neighbourhoods, represented by single-floor compact buildings between party walls. H5 is an isolated single-family house in an open green environment of the low-density building to the East of the city. B alludes to apartments in block buildings with ground floor, 1st and 2nd floor without elevator. T refers to apartments in high-rise buildings, Santa Rosa’s downtown (Fig. 5).
Wright [11], described how the use of energy at home in the UK results from a complex interaction between building style, location, energy use, occupants, and fuel affordability. Standard occupancy models predict that energy use is directly/closely related to size and shape, yet household surveys show only weak correlations in all housing types. Low-income households are grouped into five clusters based on qualitative criteria and the following variables: indoor temperature and its variation, income, surface area, envelope quality, number of family members, building age, use of oil or gas, and annual energy consumption [20-22]. In their work, authors described a model to define the number of variables according to m = log (n) / log (2) being m the number of variables to consider and n the number of cases to study.
According to the authors, in order to find the synthesis between a complex interrelation of variables in a number of case studies, statistical analyses, like regression analysis and typological analysis of clusters, are a valuable tool to understand the influence of variables. Fig. (6) shows a methodological diagram that synthesizes the analysis approach.
Quantitative analyses of measurement of indoor conditions and the calculation of heat transmission, morphological index with qualitative aspects of the users' lifestyles and customs (through interviews) were integrated. Morphological and dimensional variables (independent variables) were studied statistically through multiple linear regression analysis, allowing the relationship between a dependent variable (energy consumption) and a set of independent variables. In the multiple linear regression analysis, the corresponding equation was formulated by selecting variables.
Our aim was to find, among all possible explanatory variables, those that explain the dependent variable. The Statgraphics Plus 5.0 software was used to carry out statistical analysis.
3. RESULTS
3.1. Comparative Synthesis of the Results of Thermal - Energy Monitoring and Performance of Case Studies
Table 1 shows the synthesis of thermal and morphological characteristics. Study cases were monitored and evaluated under real conditions of use, and the performance of each case was described in previous works [23-26]. Table 2 reviews the natural gas consumption for heating during 1996-2009, together with the average temperature conditions measured during 2003-2010. It is important to note that, from a climatic point of view, the annual temperature variation, recorded during this period, was 3%, while the inter-annual variation found during winter months only was higher (10%). However, in order to simplify the analysis, pre-set temperature values in winter were used as a constant. Therefore, it allows for taking values resulting from different registration periods. In order to understand the occupant’s interactions with their built environment, a brief description of occupancy behavior, use of heating appliances and perceived comfort based on unstructured interviews are displayed in Table 3.
3.2. Single-Family Dwellings (H)
The envelope’s thermal transmittance of single-family dwellings is greater than the one recommended in a study [19]. The volumetric coefficient of loss, G, exceeds the recommendations in a study, [26] suggesting a value between 1,587 and 2.6 according to volume and heating degree-day (Table 1). The exposure factor is higher in H2 (73%) by having its west-oriented wall exposed to the outside. The compactness index varies between 57 and 73%. H5 shows little compactness (Ic = 63%) and high exposure factor and Envelope/floor area factor FAEP, 1 and 3.7, respectively.
The monitoring results of H1 were between 13-20 July 2010. This period was cold with outside temperatures that reached -5ºC. The average indoor temperature (19.9°C) was 16.3°C above the outside average (3.6°C). Between 11-15 August 2010, in H2, the average indoor temperature was around 21.8°C, 14.1°C above the average external (7.7°C). The average indoor temperature of H3 (July 13-19, 2010) was 16.0°C, 12.4 °C above the average exterior (3.6°C). H4, house facing south, with bedrooms towards the north, was occupied by two retired people. The average indoor temperature was 17.9°C, 10.5°C above the outside (7.4°C) between July 31 and August 6, 2010. H5 dwelling was evaluated during June 2003. The average indoor temperature (20.5°C) was 11.1°C above the outside (9.4°C). According to the psychometric chart of climatic conditions, the average inside temperature in dwellings is within the comfort zone with auxiliary heating energy consumption. H2 reached the maximum level of comfort, H1 and H5 reached the normal level, and H2 and H4 the minimum, according to a study [27].
As seen in Table 2, H1 is the house that shows the lowest coefficient of variation between years in the annual consumption of NG. The values registered during July and August are slightly higher, as expected for the climate harshness of those months. H2, H3 and H4 show coefficients of variation greater than those found in H1. Both H2 and H4 show greater variation in natural gas consumption during July and August within the historical period under consideration. It is possible that the increased exposure of H2, the south orientation of the living room and kitchen-dining room in H4, the habits of owners of H4 who, within the historic period of analysis, retired and stayed more hours at home, and the owner of H2, a more sedentary retired woman, have conditioned consumption.
Energy consumption agrees with the psychometric chart that shows that auxiliary heat is required to enter the comfort zone (Fig. 2). Under less adverse weather conditions, H2 consumed 100% more energy than H1 in order to reach nearly 2°C more. On the other hand, the house has greater exposure to cold winds. It should be noted that H2 had only one user in 90m2. An earlier work [26] also showed that the daily average electricity consumption in this dwelling was even lower than the city’s average consumption. This rational use of energy was not associated with the level of income, but to the users’, who expressed to live comfortably under the conditions measured (Table 3). If compared with H1 (same period measured), it can be observed that for almost 4ºC difference in average inside temperature, a decrease in consumption was 10%. According to the Institute for Energy Diversification and Savings of Spain, an increase or decrease of indoor temperature in 2ºC can lead to/result in an energy saving of 10%. Finally, H5 has a perimeter of 39.2 m, a covered area of 48.9m2 and a volume of 127.2m3, evidencing little compactness (Ic = 63%), high Exposure factor (Fe =1) and Envelope/floor area factor (FAEP = 3.7). Its Form Factor (FF) is 1.18 and its volumetric coefficient of loss is 4.1W/m3°C, widely exceeding the recommendations of the standard [27]; which, in this case, suggests a value of 2.6W/m3 °C. As seen in Table 2, the house shows one of the highest annual levels of natural gas heating / m2 with an average daily heating energy of around 10m3 and 0.20m3/m2 the usable area (10.4 m3/inhabitant), doubling that measured in H2 (0.10 m3/m2 and 4.5m3/inhabitant). As shown above, H5 and H2 have the normal and minimum comfort level according to a study [28], respectively.
3.3. Apartments in Block Buildings (B)
Filippín et al. [25], described and analyzed the monitoring of apartments in multifamily blocks. On each floor, there are 8 units (with 1, 2 or 3 bedrooms) facing NE and SW. Dimensional-morphological and thermo-physical and energetic indicators are shown in Table 2. The buildings have an independent structure of reinforced concrete and hollow ceramic brick walls. The walls and roof have no thermal insulation, and thermal transmittance does not meet the requirements [19]. The FAEP value increases toward the upper floor. Thus, the Global Loss Coefficient ‘G’ partly defined by the thermal resistance of roofs varies between 2.46 and 2.76W/m3 °C and shows higher values than those considered acceptable [27]. On the basis of the analysis of indoor temperature and natural gas consumption during the monitoring period of July-August, the apartment 12 (facing north with its single bedroom facing northwest and with its balcony closed with transparent glass carpentry) showed an average temperature of 21.2ºC (Table 2). In this apartment, the halogen heater was switched on 6h a day (between 9 p.m. and 2 a.m.). Natural gas consumption was 0.13 kWh/day/m2. Apartment 15, located at the intermediate level block in the SE corner, consumed 0.74 kWh/day/m2 (with an average temperature of 22.2°C). Apartment 23, located in the upper level (above apartment 15) with higher energy loss, consumed 0.84 kWh/day/m2 and had an average temperature of 23.7°C. Apartment 18, also on the top floor, and facing north, in the central area with its unique bedroom facing northeast, consumed 0.80 kWh/day/m2 with an average temperature of 22.3ºC. Finally, apartment 23, with the input of transferred heat from the lower flat but with greater thermal loss through its envelope, showed the largest consumption per m2. The balcony’s carpentry enclosure of apartment 12 enabled a significant reduction in energy consumption, improving comfort conditions during winter. Apartments reached the maximum level of comfort [28]. Following the psychometric chart of climatic conditions, the average inside temperature is within the comfort zone with auxiliary heating energy consumption.
3.4. Apartments in Tower Building (T)
The thermal-energy behavior of a multifamily tower building is studied [26]. The building has two blocks of 11 floors each; each ground has five apartments: A, B, C, D and E. Part of the façade of apartments A and B in the block faces north; part of the façade of apartments C, D and E in the back-block faces south, towards the block’s center (Fig. 4). The technology of T is conventional and commonly used in the country: independent reinforced concrete with a vertical and horizontal envelope without thermal insulation. Aluminum windows with PVC roller shutters, most of them with single glass, as part of the building. Walls and roofs have no thermal insulation, and thermal transmittance does not meet the requirements [19]. Table 3 shows the inside temperature evolution during 15-31 July 2009 of four apartments located on the 11th floor with mechanical heating and different orientations. In this period, the outdoor minimum temperature reached -5°C, with an external average of 6.3°C. Thermal monitoring during July allowed seeing/determining the influence of orientation and solar resource, in addition, to assess whether inhabitants lived in comfort conditions. Four monitored apartments reached welfare conditions with an average temperature of 20.5, 20.6, 23.9 and 22.4°C, respectively. Following the psychometric chart of climatic conditions, the average interior temperature is within the comfort zone with auxiliary power consumption, reaching the maximum level of comfort as per requirement [28].
It is shown that apartments D and E, facing South and SW, respectively, achieved the highest average weighted temperature: 23.9 and 22.4°C with daily natural gas consumption in heating per square meter of usable area of 0.8 and 2.2 kWh/m2/day. The least energy consumption was found in apartments B and C, with 0.6 kWh/m2/day and 0.7 kWh/m2/day, respectively, with an average weighted temperature of 20.5°C in apartment B and 12.8°C in C. Surely, the sun rays from the north produces a different thermal sensation, allowing inhabitants to live comfortably with lower temperature, and consequently, with lower energy consumption.
3.5. Analysis According to Inhabitants per Dwelling
To understand the interactions of inhabitants with the built environment, the present analysis was carried out according to inhabitant and availability of useful area per dwelling. These factors deserve their specific analysis since they can mislead the interpretation of quantitative results. In La Pampa, according to the national census, 2.97 inhabitants live per household. Only two of the households register a population of 4 inhabitants (typical family in Argentina). Case studies averaged only 1.85 inhabitants. In addition, in the case studies, most exceeded the minimum surface area suggested by the standards by 30% or more. Of the 13 cases monitored (Table 2), two exceeded by 150% the area of 38 m2/person that we have considered as an area used commonly and B18 is overcrowded with only 17m2/person.
H1 and TB have four inhabitants, with an availability of 35.5 and 32.2 m2/inhabitants, respectively. Dimensional indicators are similar (Table 1). Main differences involve the square meters of envelopes, hence, the values of FAEP are markedly different (H1= 1.53; TB = 0.64). H1 and TB reached the maximum level of comfort as per requirement [28]. According to the psychometric chart of climatic conditions, the average interior temperature is within the comfort zone with auxiliary power consumption. The ΔT indoor-outdoor is +2°C in H1. It should be noted that the energy savings according to average historical natural gas consumption achieved by TB was 15%.
H3, H4, H5, B18 and TE have 2 inhabitants per dwelling. H5 has a G-value much higher than that of the rest of the dwellings (4.10 compared to 2.70 and 3.05). The morphological indicator presents an FAEP 3 times more than the rest. The value of this index, which shows the high exposure of H5, is evidenced here. It is interesting to note that TE, whose area doubles that of H5 and its FAEP is half compared to B18, having the same orientation, shows a similar average of historical heating natural gas consumption (120-122kWh/m2/year). Even with the greater area to heating, the FAEP value seems to be a determining factor in consumption. H4 showed 15% more energy for heating than TE, as evidenced in Table 2. It can be said that facing a bad orientation (south, cold winds), the tower department is more efficient with a considerable decrease of exterior envelope. Main/Major differences lie in the square meters of the envelope, thus, the values of FAEP are largely different.
H2, B15, TC and TD have 1 inhabitant per dwelling. In this case, a comparison of the difference in terms of m2 per inhabitant should be underlined. On the one hand, H2 and TC far exceeded the local estimate of 3 m2/person (H2 = 90 m2 and TC =100 m2). Both have the same unfavorable orientation (SW and SE, respectively), equal to G-value and ΔT (Tables 1 and 2). However, the energy heating consumption is particularly dissimilar, where H2 consumes almost four times more than TC in kWh/m2/year. Although the comfort requirements differ since the TC user is in activity and, therefore, does not stay as long at home, compared to H2 who is a retired woman and stays all day in the house (Table 3). On the other hand, we compared B15 and B23 which shared surfaces, orientation and type of construction as well as inhabitants’ lifestyle. The difference in G-value and energy consumption can be attributed to the fact that B23 is in the upper floor with its roof facing the outdoor and a T+1.5C higher than B15.
Regarding the average of energy heating consumption, multifamily buildings (96.3 and 95.4 kWh/m2/year) consume /use 52% less energy than the average single-family dwellings (186.4 kWh/m2/year), under the maximum, normal or minimum level of comfort [29].
3.6. Integration of Contextual and Social Drivers According to Fig. (1) Through the Statistical Analysis
3.6.1. Regression Analysis
The output shows the results of adjustment to a multiple linear regression model to describe the relationship between heating energy consumption, dependent variable and 11 independent variables (Area, AZIMUT, ΔT (ºC), Envelope, FAEP, G-value, Heating energy consumption, Ic, Rooms, Members, Volume). The equation of the fitted model is given in Eq. (1):
y = 43.846 + 64.8495*FAEP (1)
Since the p-value in the ANOVA table is less than 0.05, there is a statistically significant relationship between variables for a 95% confidence level. The R-squared statistics indicates that the model accounts for 43% of the variability of energy consumption. To decide the simplification of the model, the highest p-value in the independent variables is considered to be 0.0150, belonging to the FAEP indicator, statistically significant for a 95% confidence level. The research done for this project involved dimensional, morphological, thermo-physical, and user indicators, a very good association was found between energy consumption for heating and FAEP in all typologies evaluated. The FAEP indicator relates envelope and useful surface. When “2” shows closer to the FEAP, envelope and exchange surface will be lower, as well the constructions cost Esteves and Geraldi [30].
3.6.2. Typological Analysis
Filippín, Ricard & Flores Larsen [31] evaluated the heating energy consumption patterns in the residential building sector using stepwise selection and multivariate analysis. The authors [32] described a retrospective analysis of the energy consumption of single-family dwellings in central Argentina and retrofitting and adaptation to climate change. In view of this, in the present work, a first analysis is made with all the variables: Useful area, Azimut, ΔT (°C), Envelope, FAEP, G-value, Heating energy consumption, Ic, Rooms, Number of household members and volume. We considered 3, 5 and 7 groups. Fig. (3), Tables 4 and 5 show the group/classification, the percentage of members in each group and the centroids of each variable. Figs. (3 to 6) show the three clusters or groups of observations with similar characteristics. 84.6% of the cases correspond to Group 1 (11 cases). Groups 2 and 3 correspond to one case each. Group 2 corresponds to H5 (single-family dwelling without walls with all its enclosure exposed to the outside environment) with an FAEP value of 3.7, north orientation, with the largest available area/member (101.4 m2), the highest G-value, and as a result, the higher consumption of energy used for heating the whole sample with the lowest Delta T (274.5 kWh/m2/year). Group 3 corresponds to the department D of group T. In this case, the lowest FAEP value of the sample (0.46), south-facing department, with an area available per user (41.5 m2/member) corresponds to this case. Even with a larger Delta T, the housing unit has the lowest energy consumption in heating (87.3 kWh/m2/year). The result confirms that within the morphological and dimensional indicators, FAEP, is the most significant of the energy consumption.
A second analysis was conducted with four variables according to the model described in a study [33] for 13 case studies: ΔT, FAEP, Heating energy consumption, Number of household members. The analysis was conducted with 3, 5 and 7 groups (Figs. 7 and 8). Table 6 shows different clusters and their members and centroids. In the three groupings, the house with a total envelope in contact with the outdoor environment consequently with a high FAEP (H5) is kept apart, as well as H2. The figure with five groups keeps the integration of H1 (single-family dwelling and apartment B of the tower building, both housing units with 4 members).
The statistical analysis that integrates social and contextual drivers allows us to observe that with 11 or 4 variables, the desegregation of the detached house with high exposure to the external environment and the higher heating energy consumption (327.3 kWh/m2/y) continues. The value of its FAEP indicator (3.7) determines an inefficient energy envelope according to [31]. The relationship between energy consumption for heating and FAEP is strong; the analysis showed a statistically significant relationship with a very high level of confidence. In this context, two proposals arise. On the one hand, the rehabilitation of the envelope was developed from the approach to build habitat to achieve a stable indoor thermal environment and reduce building energy consumption. On the other hand, the implementation of design strategies was centered on low and very low-income housing schemes [34]. The authors maintained that the development of passive low-energy buildings solves the problem.
Case Studies | Morphological and Dimentional Indicators | Energetic and Thermophilic Indicators | |||||||||||
Area | Volume | Envelope | Ic | FAEP | Azimut | G-value | U wall | U window | U roof | ||||
m2 | m3 | m2 | W/m3°C | W/m2°C | |||||||||
H Dwellings | H1 | 142.00 | 370.00 | 217.44 | 0.57 | 1.53 | N | 2.69 | 1.88 | 2.79 | 1.62 | ||
H2 | 89.70 | 172.00 | 127.50 | 0.73 | 1.42 | SW | 2.51 | ||||||
H3 | 79.80 | 224.00 | 112.00 | 0.71 | 1.40 | W | 3.00 | ||||||
H4 | 81.00 | 219.00 | 150.40 | 0.70 | 1.86 | S | 3.05 | ||||||
H5 | 48.90 | 127.20 | 149.90 | 0.63 | 3.70 | N | 4.10 | 2.21 | 5.82 | 1.62 | |||
B Apartment Block | 126 | 15 | 52.00 | 130.50 | 35.40 | 0.78 | 0.70 | SE | 2.55 | 1.84 | 2.79 | 3.82 | |
18 | 38.30 | 95.00 | 54.70 | 0.85 | 1.50 | NW | 2.76 | ||||||
23 | 52.00 | 130.50 | 85.60 | 0.78 | 1.70 | SE | 2.89 | ||||||
374 | 12 | 45.30 | 95.00 | 18.20 | 0.85 | 0.50 | NW | 2.40 | |||||
T Apartments of Tower Building | A | 76.33 | 198.47 | 56.67 | 1.42 | 0.75 | N | 2.70 | 1.84 | 2.79 | 3.82 | ||
B | 129.00 | 335.40 | 83.00 | 1.26 | 0.64 | N | 2.60 | ||||||
C | 100.40 | 261.12 | 77.12 | 1.20 | 0.77 | SE | 2.61 | ||||||
D | 41.50 | 107.90 | 19.07 | 3.11 | 0.46 | S | 2.27 | ||||||
E | 76.33 | 198.47 | 56.67 | 1.42 | 0.75 | SW | 2.70 |
Case Studies | m3(historical average periods) | Heating Energy Consumption | Measurement Period | Temperature (C°) | |||||||
(a mᶾ = 8400 Kcal) | (67 % of the annual consumption) | ||||||||||
Average | STD | CV | kWh | Kwh/m2/ year | Outside | Inside | ∆T | ||||
H | H1 | 2370.4 | 154.1 | 6.5 | 15475 | 91.5 | July - 13 July 19, 2010 | 3.6 | 19.9 | 16.3 | |
H2 | 2606.9 | 271.1 | 13.5 | 17019 | 327.3 | August 11 -August 15, 2010 | 7.7 | 21.8 | 14.1 | ||
H3 | 1178 | 163.6 | 13.9 | 7690 | 97.3 | July 13-July 19, 2010 | 3.6 | 16 | 12.4 | ||
H4 | 1417.7 | 185.2 | 13.1 | 9255 | 141.3 | July 31-August 6, 2010 | 7.4 | 17.9 | 10.5 | ||
H5 | 2056 | 205.6 | 10.0 | 13422 | 274.5 | June 4-June 18, 2003 | 9.4 | 20.5 | 11.1 | ||
B | 126 | 15 | 956.7 | 152 | 15.9 | 6245 | 120.1 | May 1 - June 29, 2009 | 10.8 | 22.2 | 11.4 |
18 | 708.8 | 76.1 | 10.7 | 4628 | 120.8 | May 1 - June 29, 2010 | 10.8 | 22.3 | 11.5 | ||
23 | 1150.8 | 101.4 | 8.8 | 7512 | 144.5 | May 1 - June 29, 2011 | 10.8 | 23.7 | 12.9 | ||
374 | 12 | 99.5 | 12 | 12.1 | - | - | May 1 - June 29, 2012 | 10.8 | 21.2 | 10.4 | |
T | A | 1707.7 | 102.2 | 16.7 | 11148 | 125.3 | - | - | - | - | |
B | 1538.2 | 115.6 | 13.3 | 10042 | 77.8 | July 15 - July 31, 2009 | 6.3 | 20.5 | 14.2 | ||
C | 1005.5 | 64.4 | 15.6 | 6564 | 63.1 | July 15 - July 31, 2010 | 6.3 | 20.6 | 14.3 | ||
D | 628.2 | 55.6 | 11.3 | 4101 | 87.3 | July 15 - July 31, 2011 | 6.3 | 23.9 | 17.6 | ||
E | 1310.5 | 95.7 | 13.7 | 8555 | 122.2 | July 15 - July 31, 2012 | 6.3 | 22.4 | 16.1 |
Case Studies | No of People | Description According to Conditions of Use | |
Occupancy Patterns /Behavioral Aspects | |||
H | H1 | 4 | Food cooking and heating is carried out by gas and/or microware oven in a multi-purpose room. The owner take showers in the bathroom located inside the multipurpose room, causing the temperature to rise during several days of the monitored period. |
H2 | 1 | The owner is a retired woman, who spends most of the time at home (having other requirements). She lives alone during the week, and in sporadic weekends her two granddaugthers sleep in the bedroom. During weekdays, this room serves as a gym from 10 at 12am. A 5000 K cal gas heater located in the living-room runs 6 h on maximun and 18 h to a minimum. While the 3000 k cal gas heater of the hall runs 6 h on maximum and 4 h to a minimum and stays 14 h on pilot. | |
H3 | 2 | Both the daily consumption of gas and electricity is low. The rational use of energy is linked not to the level of income of the user, but to the idiosyncrasies of them, who demostrated to live comfortably under these conditions. | |
H4 | 2 | A 2300 Frig Air split conditioner located in the bedroom whose outdoor wall faces east has an average daily use in summer of approximately 1 h. The dwelling is inhabited by two elderly, retired and sedentary women. A halogen heater located in the living room is operated daily for 2h. | |
H5 | 2 | A natural gas heater (4000 Kcal/h) installed between the corridor and the bathroom, was always on during the monitoring period. The dweller reported the following gas consumption schedule: (a) bumers (hotplates): 2 h/day: 2000 Kcal (b) oven: 1 h/day: 4000 kcal, (c) water heater: 0.5 h/day: 2500 kcal, and (d) air heater to maximum power: 24 h/day: 108,000 Kcal. | |
B | 126 | 1 | The owner is a retired woman, who lives there since the blocks were inaugurated (end of the 60s). The apartment has a gas heater (6000 Kcal) in the dining room which is turned off in the absence of the owner. The heater is on for 6 h a day from 10 am. The dining-room is mostly used as a workplace, computer room and reading area. |
2 | The apartment has a 6000 Kcal gas heater in the dining-room, which is kept on during 6 h a day to the minimum. The heater is swicted off when it is no occupied. | ||
1 | The owner, an elderly woman, lives there since the end of the 60s when the blocks were inaugurated. The apartment has two gas heaters, one in the dining room and the other in the internal bedroom. The bedroom heater is on for about 6 h a day and the one in the dining room is on for about 4 h a day from 10pm. | ||
374 | 2 | The apartment does not have a gas heater. The dweller uses a 1200W oscillating halogen heater (three levels = 400-800-1200) that is turned on during 1 h in the morning and between 9 pm and 2 am approximately in the evening/night). | |
T | A | - | Not Monitored. |
B | 4 | This apartment is occuped by a standard family with a couple and two kids. | |
C | 1 | The owner is a single middle aged man. | |
D | 1 | Thw owner is a retired woman who lives alone. | |
E | 2 | The apartment was not occupied until July 18 at midday. From that moment onwards, heaters were turned on, increasing the indoor temperature to 25 C; the temperature did not go beyond 22.°C nor under 17 °C. The effect of tuming the heater on during night is noticeable, which, on average, increases the indoor temperature by 2.5 °C. |
Cluster | Members | % | Cluster | Members | % | Cluster | Members | % |
1 | 11 | 84.62 | 1 | 2 | 15.38 | 1 | 1 | 7.69 |
2 | 1 | 7.69 | 2 | 1 | 7.69 | 2 | 1 | 7.69 |
3 | 1 | 7.69 | 3 | 8 | 61.54 | 3 | 7 | 53.85 |
– | – | – | 4 | 1 | 7.69 | 4 | 1 | 7.69 |
– | – | – | 5 | 1 | 7.69 | 5 | 1 | 7.69 |
– | – | – | – | – | – | 6 | 1 | 7.69 |
– | – | – | – | – | – | 7 | 1 | 7.69 |
Cluster | Area | AzimutT | Δ T (°C) | Envelope | FAEP | G-value | Heating | Ic | Rooms | Members | Volumen |
1 | 80.53 | 135.00 | 13.10 | 92.54 | 1.16 | 2.70 | 118.73 | 0.89 | 2.09 | 2.00 | 202.81 |
2 | 48.90 | 180.00 | 11.10 | 149.90 | 3.70 | 4.10 | 274.50 | 0.63 | 1.00 | 1.00 | 127.20 |
3 | 41.50 | 0.00 | 17.60 | 19.07 | 0.46 | 2.27 | 87.30 | 3.11 | 1.00 | 1.00 | 107.90 |
1 | 135.50 | 180.00 | 15.25 | 150.22 | 1.08 | 2.64 | 84.65 | 0.91 | 3.00 | 4.00 | 352.70 |
2 | 89.70 | 45.00 | 14.10 | 127.50 | 1.42 | 2.51 | 327.30 | 0.73 | 2.00 | 1.00 | 172.00 |
3 | 65.64 | 135.00 | 12.43 | 73.76 | 1.15 | 2.74 | 101.18 | 0.91 | 1.87 | 1.62 | 169.19 |
4 | 48.90 | 180.00 | 11.10 | 149.90 | 3.70 | 4.10 | 274.50 | 0.63 | 1.00 | 2.00 | 127.20 |
5 | 41.50 | 0.00 | 17.60 | 19.07 | 0.46 | 2.27 | 87.30 | 3.11 | 1.00 | 1.00 | 107.90 |
1 | 142.00 | 180.00 | 16.30 | 217.44 | 1.53 | 2.69 | 91.50 | 0.57 | 3.00 | 4.00 | 370.00 |
2 | 89.70 | 45.00 | 14.10 | 127.50 | 1.42 | 2.51 | 327.30 | 0.73 | 2.00 | 1.00 | 172.00 |
3 | 60.67 | 122.14 | 12.17 | 73.28 | 1.20 | 2.76 | 106.63 | 0.87 | 1.71 | 1.71 | 156.07 |
4 | 48.90 | 180.00 | 11.10 | 149.9 | 3.70 | 4.10 | 274.50 | 0.63 | 1.00 | 2.00 | 127.20 |
5 | 129.00 | 180.00 | 14.20 | 83.00 | 0.64 | 2.60 | 77.80 | 1.26 | 3.00 | 4.00 | 335.40 |
6 | 100.40 | 225.00 | 14.30 | 77.12 | 0.77 | 2.61 | 63.10 | 1.20 | 3.00 | 1.00 | 261.12 |
7 | 41.50 | 0.00 | 17.60 | 19.07 | 0.46 | 2.27 | 87.30 | 3.11 | 1.00 | 1.00 | 107.90 |
Cluster | Members | % | Cluster | Members | % | Cluster | Members | % |
---|---|---|---|---|---|---|---|---|
1 | 11 | 84.62 | 1 | 2 | 15.38 | 1 | 1 | 7.69 |
2 | 1 | 7.69 | 2 | 1 | 7.69 | 2 | 1 | 7.69 |
3 | 1 | 7.69 | 3 | 6 | 46.15 | 3 | 5 | 38.46 |
– | – | – | 4 | 1 | 7.69 | 4 | 1 | 7.69 |
– | – | – | 5 | 3 | 23.08 | 5 | 1 | 7.69 |
– | – | – | – | – | – | 6 | 1 | 7.69 |
– | – | – | – | – | – | 7 | 3 | 23.08 |
Cluster | ΔT (°C) | FAEP | Heating | Members |
1 | 13.41 | 1.07 | 96.91 | 2.00 |
2 | 14.10 | 1.42 | 327.30 | 1.00 |
3 | 11.10 | 3.70 | 274.50 | 1.00 |
1 | 15.25 | 1.08 | 84.65 | 4.00 |
2 | 14.10 | 1.42 | 327.30 | 1.00 |
3 | 11.51 | 1.27 | 104.03 | 1.66 |
4 | 11.10 | 3.70 | 274.50 | 1.00 |
5 | 16.00 | 0.66 | 90.86 | 1.33 |
1 | 16.30 | 1.53 | 91.50 | 4.00 |
2 | 14.10 | 1.42 | 327.30 | 1.00 |
3 | 11.74 | 1.43 | 124.80 | 1.60 |
4 | 11.10 | 3.70 | 274.50 | 1.00 |
5 | 10.40 | 0.50 | 0.20 | 2.00 |
6 | 14.20 | 0.64 | 77.80 | 4.00 |
7 | 16.00 | 0.66 | 90.86 | 1.33 |
3.7. Architectural Proposal Considering the Use of Resources
Santa Rosa finds itself at a crucial moment in its unplanned historical growth, showing an imbalance in center-periphery density. The strategic plan for the development of Santa Rosa [35] establishes:
- To increase the density in the consolidated central area and to minimize the area of idle land.
- To optimize the consumption of energy according to its different uses.
- To contribute to the complexity of medium-density areas (between 20 and 50 homes per hectare) and to promote typologies of collective housing in 3 or 4 floors, surrounded by open spaces that facilitate nature and recreational activities.
- To regroup homes into a compact design of single volumes, simple and compact, involves ecological and economic strengths (eight units in a multi-family building with three floors reduce land occupation by 66%, compared to an isolated house which reduces the number of materials, infrastructure, etc.).
- To discourage the growth of very-low-density areas (from 4 to 10 houses per hectare).
Based on the comparative synthesis and integration of contextual and social drivers through statistical analysis, an architectural proposal that incorporates the use of resources and energy efficiency strategies is theorized. The proposal must satisfy the total housing deficit: 4300 families, the lowest value in Argentina [36]. The goal is to give an answer to the mitigation of the dwelling deficit and the energy poverty [22]. The authors define the equation Fuel Poverty = energy consumption*Price/Income. According to this equation, the alternative to reduce energy consumption and the energy poverty of the most vulnerable sectors with lowest income is to intervene in urban planning and architectural design. The proposal recommends H and B located in different parts of the city where there is good connectivity and public transport infrastructure and services. These typologies have been selected on the basis that none requires elevators and high maintenance costs [37].
The design, which is energy-efficient, healthy and environmentally sustainable, is associated with the morphological and dimensional indicators from the results of the analysis described. Thus, and in the next phase of this research, design measures are being proposed to reduce the housing deficit and energy poverty, preserving resources.
These examples (Figs. 9 and 10) derive from a logical conclusion following the synthesis analysis as shown in Fig. (5), and seeks to meet the first objective of this article to handing in an architectural proposal considering the use of resources (land, morphology, materials and energy), the lifestyle of the inhabitants (qualitative variables) and the critical analysis of Argentina’s historical problem of housing deficit. Fig. (9) and 10) show different possible sites of the new neighbourhoods with pre-existing infrastructure and service. Proximity and connectivity to main roads, public and private transport have been considered. Fig. (10) shows free land within the urban grid. This is only part of the city; the northern zone, one of the most consolidated one. The strategic plan imposes sanctions for idle urban land. It is important to consider that part of the void land comprises urban barriers like unused railway land and borders of the ring road. The eastern border of the city also represents a great/favourable opportunity for land use with infrastructure and services.
Analysing the housing requirements for the exercise, some aspects must be defined. On the one hand, and according to a study [38] 2.9 inhabitant/dwelling relation [8] was considered to build 60m2/family, reaching a total construction of 258000 m2 without galleries or pergolas. On the other hand, complexes of single-family dwelling built by the provincial state in 2005 showed 40 dwelling/ha., and complexes of multifamily dwelling built with loans from a national bank during 1960-1970 showed an 80-96 dwelling/ha rate. Hence, for single-family dwellings (typology H), land could be between party walls of 8 x 20 m depth arranged in city blocks of 170 m and 50 m depth (including the corresponding street surface and sunlight access). In order to this 0,85ha. each city block comprises 40 single-family dwellings aligned by two that help to decrease vertical envelope (Figs. 11 and 12). Plus, the land has convenient N-S orientation, both fundamental bioclimatic strategies. In this case, 90,3 ha would be required for all families.
We propose 2 types of B, including the corresponding street surface (Fig. 12): one of 110 x110 m and another of 50 x170 m with 1.26 ha each block. In this case, 38ha would be required for the 4,300 families divided into 30 blocks with 144 families each. The building has ground floor, 1st and 2nd floor without elevator and 8 apartments of 60 m2/floor. By comparing H with B, H uses 58% more of land than B. This is compensated with inhabitant’s access to fertile soil for mini-orchard cultivation and fruit trees, greater privacy, less noise, outdoor areas and the possibility of expanding areas on the ground floor and in height to adapt the design as per the family requirements [39]. Each family in H owns 200m2 of land, whereas those in B only own 88.4 m2 (North to top).
Considering the recommendations [30] and Sulaiman, Mazzocco and Filippín [40], the height of the vertical envelope has a significant impact on the cost of construction and energy use for acclimatization. An interior height of 2.50 m (2.4 m is the minimum for social houses) is thus proposed here. In agreement with the same authors, the FAEP value is 1.46 and 0.8 for H and B, respectively (smaller enclosure area per m2 of useful area). It is important to note that the real envelope does not comply with the hygrothermal conditions of current national regulations.
The strategy of energy conservation is technologically feasible and it would allow achieving thermal transmittance values in accordance with the relevant IRAM recommendation. In winter [19], the study recommends a U-value for walls of 0.30 and 0.80W/m2 °C, levels A and B, respectively, according to an outside design temperature of -6°C. Thus, a U-value of 0.47W/m2 ºC can be obtained with the addition of a 0.05m thick layer of extruded polystyrene to the exterior protected with coat base, a cementitious material with additive polymer. The standard also recommends a U-value for roofs 0.26 and 0.67W/m2 ºC, levels A and B, respectively. Thus, a U-value of 0.38W/m2 ºC can be obtained with the addition of a 0.075 m thick layer of extruded polystyrene to the exterior facing roof. Double hermetic glazes and external shutters reduce the U-value around 1.05W/m2 ºC. In agreement with the thermal improvement of the entire enclosure, energy loss decreases in both types. Table 6 summarizes all the factors considered in this proposal. The convenient orientation of all H dwellings would promote good energetic behaviour despite having greater external envelope than B. The B dwelling with S orientation, half of the total, has the possibility of accessing natural light with technology available in the market like [41]. In relation to energy saving and, according to the equation [2] provided in the present work and to the FAEP of each typology, heating energy is around 138.4 and 94.4 kWh/ m2/year for H and B, respectively. Consequently, energy saving is 32%, a promising value for a city that imports energy and depends on natural gas in winter (67% of the total annual consumption of the fluid is mainly required for the heating of homes). Regarding the access of natural light (availability of natural daylight) for the illumination of the interior space, both types can use different design components. The southern sector of B could allow entry of natural light from the roof through tubular daylighting (technology available in Argentina). Therefore, the availability of the solar resource in order to heat and illuminate the spaces, in addition to an adequate use by inhabitants of the strategies and design components, would allow reducing energy consumption.
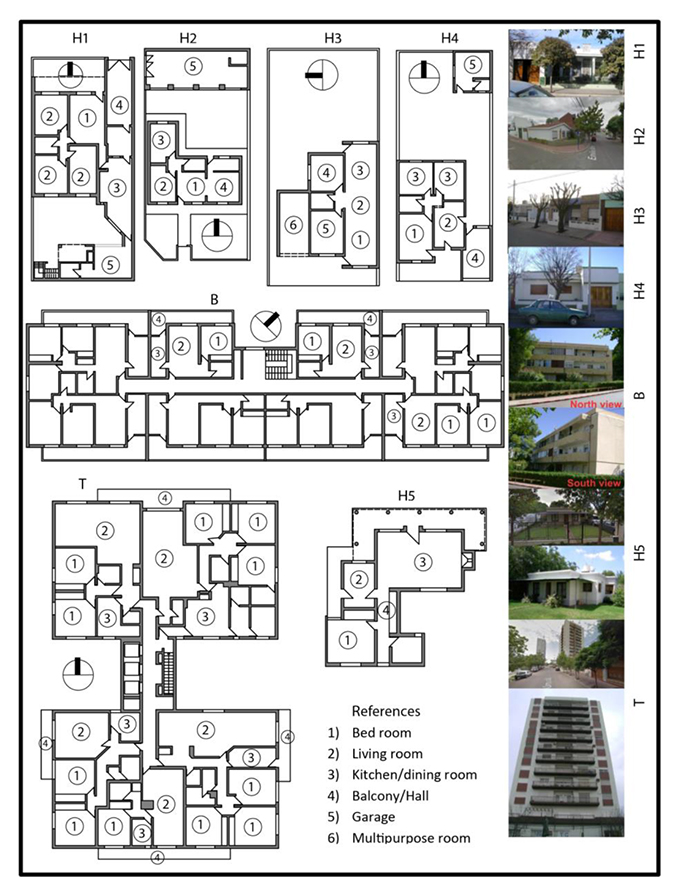
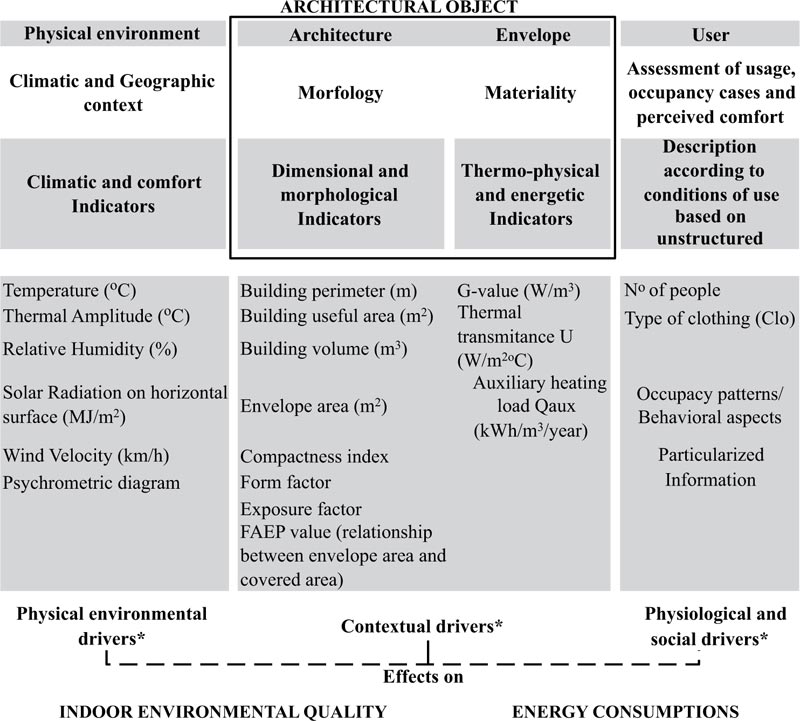
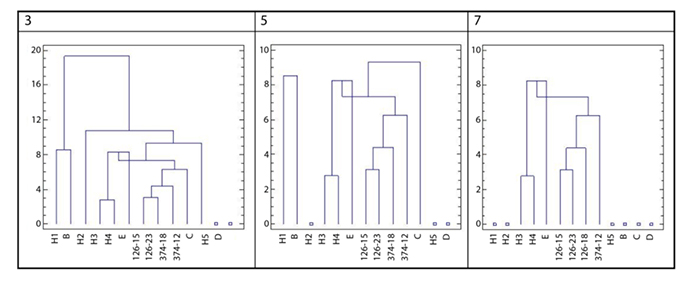
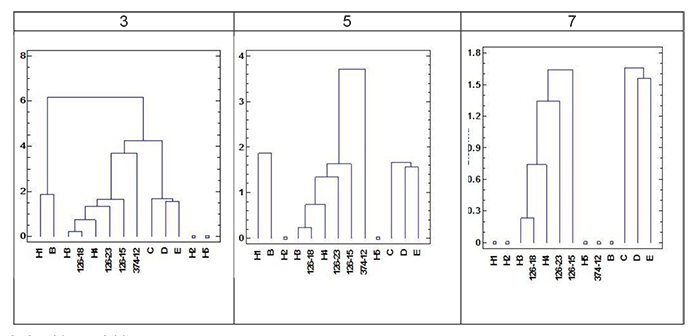
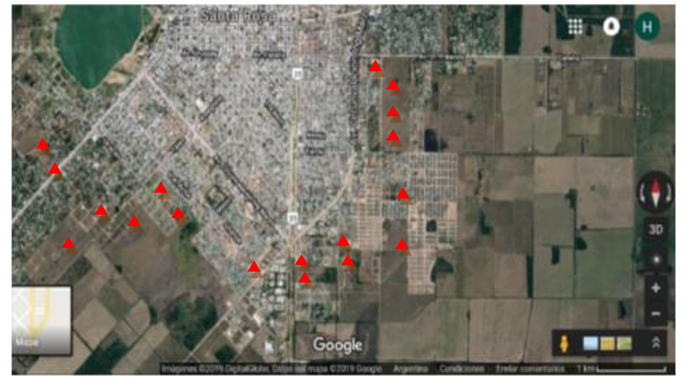
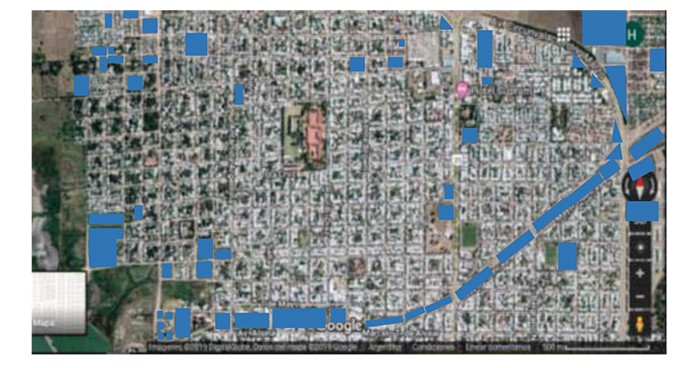
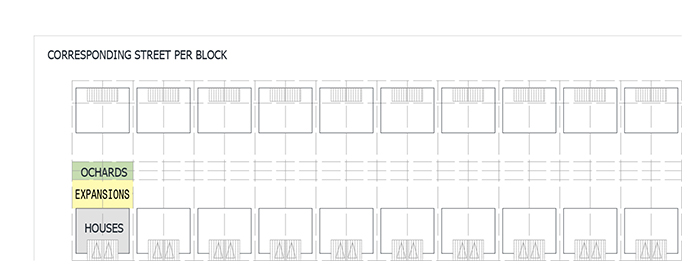
Rettrofiting Approach for 4300 Families | |||||||
---|---|---|---|---|---|---|---|
Contextual Drivers | H | B | Efficiency (%) | ||||
Land | Total | hectare | 90.3 | 38 | 58 | ||
Per family | 0.021 | 0.0088 | |||||
Morphology and Materiality | U wall | W/m2ºC | 0.67 | ||||
U window | 1.05 | ||||||
U roof | 0.58 | ||||||
Vertical isolated envelope | m2 | 126688 | 104328 | 18 | |||
Isolated roof | 249400 | 96750 | 61 | ||||
Thermal losses through | the vertical envelope | W/ºC | 84881 | 69900 | 18 | ||
the roof | 144652 | 56115 | 61 | ||||
FAEP | 1.46 | 0.8 | 47 | ||||
Energy Saving | Energy heating | kWh/m2/year | 138.4 | 94.4 | 32 | ||
Availability of natural daylight | % | 100 | 100 | - | |||
Qualitative Variables | Orchad (No interpreto) | Individual | Community | ||||
Accessibility to leisure and recreational spaces | Yes | Yes | |||||
Privacy | Yes | No | |||||
Future Growth | Yes | No | |||||
Future division in independent units | Yes | No |
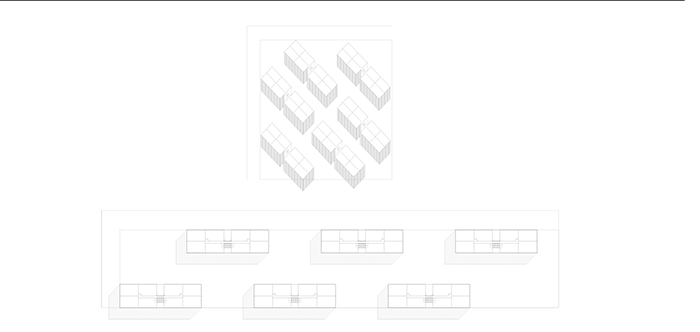
4. DISCUSSION AND CONCLUSION
The development of the work allowed fulfilling the objectives sought. We carried out a global comparative synthesis of the results of monitoring thermal energy behavior of 13 single-family and multifamily buildings. Dwellings reach the maximum level of comfort according to the study [28]. In view of the psychometric chart of climatic conditions, the average interior temperature is within the comfort zone with auxiliary power consumption. The result of the typological analysis ratifies that, within the morphological and dimensional indicators, FAEP is the most significant of energy consumption. The housing analysed shows a difference in energy consumption when compared to both typologies located in the city researched. The consumption of natural gas for the heating of an isolated house (H5) is almost 70% and 40% higher than that for an apartment of a multifamily building tower (T) and block (B), respectively. Single-family buildings (H) include compact dwellings between party walls, having 50% less natural gas consumption than the social housing in the same region (208.9 kWh/m2) [42]. It confirms the results as reported in a study [43] where the authors conclude that electricity and heating energy consumption is much higher in lower-income groups due to the construction and materials quality that they can reach.
The results of the monitoring thermal energy behavior of dwellings in the central region of Argentina suggest/indicate that the energy saving of apartments and single-family housing is greater than that reported in a study [44]. The authors claimed that the apartments in Palermo (Italy) show a saving of around 7.3% in heating energy consumption with regard to single-family dwellings. In the case of Murcia and Valladolid (Spain), the value rises to 8.9% and 24.7%, respectively. An energy analysis of homes in the Extremadura community shows that the demand for heating moves in a range between 100 and 200kWh/m2 for single-family homes, and between 60 and 150kWh/m2 for multi-family homes. According to the average, the reduction in energy consumption in multi-family buildings is around 30% [45].
An architectural proposal that considers the use of resources (land, morphology, materiality and energy), the lifestyle of inhabitants (qualitative variables) and the critical analysis of the historical problem of housing deficit suggests/indicates to be in agreement with the study [46, 47]. They affirmed that the densification of housing into simple compact volumes provides significant energy and economic benefits. Build urban environments with a building density and optimal urban compactness, guarantees the balance between the built space and the free space. The creation of different environments benefits the social cohesion of future inhabitants. The sustainable city model would be one that, by reversing the current trend, gradually reduces energy (resource consumption) while increasing the value of urban organization. In addition, if the building design implements the National Standards’ recommendations on thermal permeability of envelopes, at least in governmental building and social dwellings, the reduction of energy consumption would be achieved. A different angle [48], reports that high-rise multifamily buildings have the least environmental impact as compared to single-family housing.
CONSENT FOR PUBLICATION
Not applicable.
AVAILABILITY OF DATA AND MATERIALS
Not applicable.
FUNDING
None.
CONFLICT OF INTEREST
The authors declare no conflict of interest, financial or otherwise.
ACKNOWLEDGEMENTS
We would like to thank the inhabitants of the monitored dwellings and apartments for their generous collaboration. We are also especially grateful to Cooperativa Popular de Energía Eléctrica, Santa Rosa, together with Camuzzi Gas Pampeano for the information supplied.